Chemical Sensors
NASA is developing chemical species sensors for aerospace applications including leak detection and emission, safety, human health, and environmental monitoring.
Overview
The Chemical Species Gas Sensors Team at NASA’s Glenn Research Center in Cleveland is developing microsensing technology for aerospace applications. Their approach is to implement sensitive and selective sensor systems capable of providing quantitative measurements of the environment, enabling better cognition and decision-making. The sensors can be used for leak detection and emission, safety, human health, and planetary exploration. The resulting sensor system is meant to be small, smart, and rugged with capabilities tailored for the targeted application.
The program develops four different types of sensor platforms for chemical sensing. One platform is a Schottky diode sensor structure for use in very sensitive measurements. Low concentrations of hydrogen and hydrocarbons can be detected using this basic structure. Three other platforms—resistive-based techniques, electrochemical cells, and nanoplasmonic structures—are used depending on the species and concentration range to be detected.
Producing sensor arrays using these platforms is an ongoing activity of this group. For example, one goal of this gas sensor research is to create a microfabricated gas sensor array operable at high temperatures such as in an emissions stream. Since one sensor will not be able to characterize multiple species in such an environment, an array of high-temperature sensors is necessary.
This array, effectively a high-temperature electronic nose, is a significant step in allowing the monitoring and/or control of emissions produced by an aeronautic engine. The signals produced by this sensor array could be analyzed to determine the constituents of the emission stream.
This information could then be used to monitor changes in engine health or control those emissions. Sensor arrays have also been developed for other applications.
Sensor Platform Approach
These sensors are microfabricated using Microelectromechanical Systems (MEMS)-based technology to minimize size, weight, and power consumption. Nanostructures are used to improve the sensor response and stability and can be integrated into microstructures. A temperature detector and a heater are standardly included in the sensor structure to allow stable sensor operation at a variety of temperatures.
Mass fabrication of the sensors using silicon-processing technology is envisioned to minimize the cost per sensor. The program develops four different types of base sensor platforms. One platform is a Schottky diode sensor structure for use in very sensitive measurements. Two other platforms—resistor-based techniques and electrochemical cells—are used depending on the gas and concentration range to be detected. Another platform takes advantage of the unique capabilities provided by nanoplasmonic sensors.
These sensor platforms are foundations on which a wide range of species may be measured by choice of materials and operating parameters. For example, the electrochemical cell platform can be modified to produce an oxygen sensor or a carbon dioxide sensor depending on the electrolyte, materials, and operating temperature.
Integration into Smart Sensor Systems
The sensors developed from these platforms allow the detection of a range of species including hydrogen, hydrocarbons, nitrogen oxides, carbon monoxide, oxygen, and carbon dioxide in a variety of ambient gas conditions and temperatures. A core foundation of this work is the integration of these sensors, either individually or in a sensor array, into Smart Sensor Systems.
A Smart Sensor System, as conceived of here, is a complete self-contained sensor system that includes multiparameter sensing, data logging, processing and analysis, self-contained power, and an ability to transmit or display information.
Multiparameter information is obtained to understand the environment. Local processing is used to optimize the quality and relevance of that information, and then it is communicated in a manner that best fits the application. The approach has been to develop “Lick and Stick” technology that can be stand-alone and applied wherever and whenever necessary.
Wide Range of Applications
The application range of this technology has been broad and impactful. Applications over time have included leak detection, fire and environmental monitoring, engine emissions, human breath and exercise monitoring, and cryogenic fuel line monitoring.
The work has often involved collaborators such as Case Western Reserve University and The Ohio State University, as well as hardware development in NASA’s Small Business Innovation Research (SBIR) and Small Business Technology Transfer (STTR) program by Makel Engineering Inc.
Applications of the technology developed in this work range from the International Space Station to the surface of Venus. This page provides a sampling of the sensors, sensor arrays, and their applications.
Selected Awards
- NASA Inventors Hall of Fame (2024)
- NASA Invention of the Year Honorable Mention (2017)
- Vehicle Integrated Propulsion Research (VIPR) Group Achievement Award (2016)
- Abe Silverstein Medal for Exceptional Technical Achievement (2011)
- Outstanding Paper of the Year, Sensors Review Journal (2011)
- Nominated for NASA Invention of the Year (2009)
- Nano 50 Award for Advancement of Nanotechnology (2008)
- R&D 100 Award for Fire Detection System (2005)
- NASA Turning Goals into Reality Associate Administrators Choice Award (2005)
- NASA Turning Goals into Reality Safety Award (2003)
- R&D 100 Award for Leak Detection System (1995)
Gas Sensors
Hydrogen Sensing
In launch applications, hydrogen leaks pose significant operational safety concerns. In response, NASA Glenn developed microfabricated point-contact hydrogen sensors for finding the position of the leaks. One component of this program involves the fabrication of palladium-alloy hydrogen sensors on silicon (Si) substrates. The hydrogen sensor includes a Schottky diode and a hydrogen sensitive resistor for detection from the parts per million (ppm) range to 100% hydrogen (shown below).
The sensor includes a temperature detector and heater for operation in a wide variety of environments. It has high sensitivity and can operate in either inert or oxygen-containing environments. The operating range of the sensor can be designed from ppm to 100% hydrogen.
These sensors have been commercialized by Makel Engineering Inc. into a complete, wide range, leak detection system. They are part of a safety system on the International Space Station, have flown or been used in multiple applications, and are presently used by Blue Origin. These sensors are being considered for a future mission to Titan, Saturn’s icy moon. The basic platform structure can also be used for the detection of toxic gases such as hydrazine.
A second component of the program involves the use of silicon carbide (SiC) rather than silicon (Si) as the semiconductor in a Schottky diode structure. The use of SiC has been demonstrated for applications that require high-temperature detection of hydrogen in conditions in which Si-based technology will not function. High-temperature detection is further discussed in the Hydrocarbon Sensing Technology section.
Hydrocarbon Sensing
The detection of hydrocarbons is important in the monitoring of aeronautic engine exhaust, leak detection, and fire detection. For example, aeronautic gas emissions are often at temperatures considerably above the threshold where the silicon semiconductor hydrogen sensor is operable. Furthermore, it is at these higher temperatures that catalytic effects occur that often make hydrocarbon detection possible.
For these higher temperature applications, a Schottky diode using silicon carbide (SiC) rather than silicon (Si) semiconductor technology has been developed. The material properties of SiC make it suitable for operation in hostile conditions, which exceed the inherent limitations of Si-based electronic devices. In particular, the ability of SiC to operate as a semiconductor at temperatures higher than 600ºC makes it useful in high-temperature emission measuring applications, as well as for the development of high-temperature electronics.
Thus, the use of a SiC rather than Si semiconductor in a Schottky diode structure allows this platform to be used at high temperatures. The use of precious metal catalysts in the Schottky diode structure allows the sensor to respond to hydrocarbons with high sensitivity. After notable development, the sensor has shown stability at high temperature for long durations. Further, the same sensor structure with internal temperature control can operate in applications that are not at high temperatures but also require the need for sensitive hydrogen or hydrocarbon detection.
A SiC-based Schottky diode hydrocarbon and hydrogen sensor package including a temperature detector and heater has been applied in multiple applications including leak detection, emissions monitoring, and fire detection. Advancements in this area have been patented and were nominated for NASA Invention of the Year in 2009 and received honorable mention for the 2017 NASA Invention of the Year. Present work includes integrating modifed versions of such sensors as these with SiC-based high-temperature electronics.
Nitrogen Oxide Sensing
NASA Glenn has developed multiple methods of nitrogen oxide (NOx) detection. One approach, in a previous collaboration with The Ohio State University, is based on a solid-state electrochemical NOx sensor for breath monitoring.
The emphasis of this work was on the miniaturization of the sensor based on larger sensor technology. Due to its capability of resisting high temperatures, the sensor could also be extended to applications such as monitoring NOx from a jet engine.
Research at NASA Glenn focused on miniaturizing these sensors as part of a program with the Cleveland Clinic Foundation with funding provided by the Ohio Third Frontier program. Alternate approaches are based on SiC Schottky diodes or other electrochemical cell structures.
Carbon Monoxide Sensing
The detection of carbon monoxide (CO) is necessary for a range of applications. There is a need for improved CO sensors for fire detection and environmental monitoring. CO detection is also of interest in aeronautic emissions applications. NASA Glenn has supported the development of two major types of CO sensor technology: one based on tin oxide (SnO2), and the other based on titanium dioxide (TiO2). Both have been miniaturized for smart sensor applications.
For example, one CO sensor type uses specially processed tin oxide (SnO2) as the gas sensitive material to ensure stable operation. A standard characteristic of SnO2 is that it undergoes drift at high temperatures due to annealing of the grains. However, nanocrystalline SnO2 provides greater stability and sensitivity at higher temperatures due to its small grain size and large surface area and is thus more useful as a sensor. By doping (through the addition of impurities, such as another element) the SnO2 appropriately, the sensor can be fine-tuned to become more sensitive to either CO or NOx.
Oxygen Sensing
Multiple types of oxygen sensors have been developed using an electrochemical cell platform. This has ranged from sensors that operate at high temperature with zirconia electrolyte to one that runs at room temperature with NAFION electrolyte.
For example, a microfabricated O2 sensor has been developed for safety purposes in aerospace applications, but significant applications also exist in aeronautic emissions control and human health applications.
Conventional oxygen sensors utilize an electrochemical cell containing ZrO2, which conducts oxygen ions at high temperatures. These standard cells are bulky and power consuming. The oxygen sensors developed contain a complete microfabricated electrochemical cell mounted onto a small chip.
The main benefits of this sensor approach include a wider oxygen concentration measurement range, less power consumption, and cheaper production costs.
A room temperature version of this sensor has been developed using a different electrolyte. NAFION solution doped with water-retaining components are coated on the electrode area to form a conducting electrolyte layer. The sensor is operated under potentiometric mode, which means electrical potential difference was measured between these two electrodes.
Carbon Dioxide Sensing
Research has been on-going to create smaller, cheaper, and more accurate CO2 sensors with a wide temperature range.
Combined with the CO sensors, these devices would have important fire detection applications as well as in aeronautic applications in emissions monitoring and human health monitoring. Similar in basic approach to the oxygen sensors, these CO2 sensors make use of a solid electrochemical cell.
In this design, the solid electrolyte used is NASICON (sodium super ionic conductor). Stable and repeatable operation of this sensor has been demonstrated for significant periods of time and improved operation of the sensor has been shown by the addition of nanomaterials. This device is microfabricated to enable the benefits of lower production and operating costs.
Nanoplasmonic Sensing
Nanoplasmonic sensors exploit the phenomenon of “surface plasmon resonance”—coupled electron–electromagnetic wave oscillations a metal–dielectric interface under incident illumination by light. These resonant surface waves have confined/enhanced electric fields, which are highly sensitive to changes in the local dielectric environment, e.g., the binding of a biomolecule or chemical species. The resulting change in dielectric environment is transduced as a shift in the resonance feature. Surface functionalization is required to ensure sensor specificity, and different approaches are used depending on the target application, including polymer-based recognition elements, templated “lock-in-key”/artificial antibody approaches, and bio-affinity interactions (e.g., DNA/RNA hybridization).
A primary goal of this work is to transition this sensing technology out of the lab, where it often relies on lab-based instrumentation (microscope, spectrometer, etc.) for signal excitation and readout, to the portable, field/space-deployable systems. Different sensor geometries have been investigated, which enable integration with on-chip photonic integrated circuits, while maintaining high sensitivity and the other desirable properties of nanoplasmonic sensing.
Benefits of nanoplasmonic sensors for NASA’s mission: low-temperature operation/potential for in-situ measurements (e.g., icy moon/ocean worlds), tailorable recognition elements for a wide range of applications, real-time and label-free operation.
Sensor Arrays
Leak Sensor Array
In leak monitoring of launch vehicles (rockets that carry payloads to space), it is important to detect low concentrations of hydrogen and other fuels to avoid explosive conditions that could harm personnel and damage the vehicle. Dependable launch vehicle operation also relies on the timely and accurate measurement of these leaks. Measuring the levels of hydrogen and hydrocarbons (fuel) and oxygen can indicate whether there is an explosive condition in each region.
A MEMS-based leak detection sensor system that integrates hydrogen, oxygen, and hydrocarbon sensors into a single package has been achieved. The work creates a smart, adaptable system that has the surface area of a postage stamp and can simultaneously measure both fuel and oxygen, transmit data through telemetry, and have low power consumption.
This smart sensor package contains the required analog and digital electronics, including processing, memory, and communication devices to enable a stand-alone system. This basic hardware approach for hydrogen has been matured for potential implementation on next-generation launch vehicles. The approach is to produce a complete leak detection system that can be placed in several locations. This core smart sensor hardware can also have a range of other applications.
Fire and Environmental Monitoring
Fire detection is extremely important aboard space and commercial aircraft to avoid catastrophic situations and to verify the operational status of the vehicle. Previous false alarm rates from traditional fire detectors were caused by changes in humidity, condensation on the detector’s surface, or contamination from the contents of the vehicle.
The program’s work produced a microsensor array to measure the chemical signature of a fire to provide more reliable fire detection. The carbon monoxide and carbon dioxide sensors are central to this array, and other sensors are included to improve the accuracy of the measured chemical signature providing indications of conditions that can produce false alarms in other detectors.
Some of the same sensors are also of interest for environmental monitoring of a cabin environment. For example, carbon monoxide and carbon dioxide are also core measurements to assure a safe cabin environment, but at different concentrations than needed for fire detection. Modifications to the core carbon monoxide and carbon dioxide sensor technology provide other sensors for this measurement range. The result is a multiparameter sensor array that can be used for both environmental monitoring as well as fire detection.
This fire and environmental monitoring work has produced prototype systems that are both hand-held and stationary. More recent work has involved a prototype wearable monitor for first responders, e.g., wildfire conditions.
Engine Emissions Sensor Array
A high-temperature engine emissions gas sensor array, in effect a high-temperature electronic nose, has been developed to detect a variety of gases of interest for emissions and chemical process monitoring such as hydrocarbons, nitrogen oxides, carbon monoxide, carbon dioxide, and oxygen. Several of these arrays could be placed around the exit of the engine exhaust to monitor the emissions produced by the engine.
The signals produced by this emissions sensor array could be analyzed to determine the constituents of the emission stream, and this information can then be used to control those emissions. This emissions sensor array concept uses materials that are suitable for high-temperature environments and that are composed of different types of microsensor platforms (diodes, resistors, and electrochemical cells) to give a wider range of inputs.
This approach has been demonstrated multiple times at the exhaust of a jet engine, and the data suggests a method for correlating the measurements with the engine’s health state.
Sensor Applications
Chemical Sensors Family Tree
The approach of this group, its collaborators, and the work in the STTR/SBIR program has been to start with a core set of sample platforms combined with Smart Sensor Systems to address a large range of applications. An example of the approach is shown below with a chemical sensors family tree: one sensor platform can yield the capability to measure multiple chemical species, and the combined platforms have extended versatility.
Applications
This work has led to a vast range of applications. In each case, the chemical sensors and associated hardware have been tailored for the application. The following list outlines where this micro chemical sensor technology has either been demonstrated and applied, or where it is in development.
- Jet Engine Emissions
- Aircraft Fire Detection
- Exercise Monitoring
- International Space Station Safety System
- Launch Vehicle Engine Test Stands
- Environmental Monitoring
- Cryogenic Fuel Line Monitoring
- Launch Vehicle Leak Detection
- Human Health Breath Monitoring
- First Responder Monitoring
- Long-Lived Venus Surface Missions and Atmospheric Characterization
- Hydrogen Powered Aircraft
- Titan Atmospheric Exploration
Contact
Area of Expertise | Name | |
---|---|---|
Chemical Sensors | Gary Hunter | gary.w.hunter@nasa.gov |
Chemical Sensors | Jennifer Xu | jennifer.c.xu@nasa.gov |
Sensor Applications | John Wrbanek | john.d.wrbanek@nasa.gov |
NASA Glenn facilities where this research is conducted:
Microsystems Fabrication Laboratory
This vertically integrated silicon carbide (SiC) semiconductor research and development facility is dedicated to the design, fabrication, and testing of integrated circuit electronics and sensors uniquely durable to extreme environments.
Learn More about Microsystems Fabrication Laboratory
Key Publications
Publication Title | Author(s) | Source | Type | Year |
---|---|---|---|---|
High Temperature Smart Sensor Systems for Venus and Aerospace Applications (Invited) | Gary W. Hunter, Darby Makel, Susana Carranza, and Jennifer Xu | 18th International Meeting on Chemical Sensors, Virtual Meeting, USA, May 30th – June 3rd 2021 | Presentation | 2021 |
Critical Review of Solid State Gas Sensors (Editors’ Choice) | G. W. Hunter, C.C. Liu, P. Hesketh, S. Akbar, D. Miller, J. Stetter, C. E. Davis, R. Ramasamy, and A. R. Hillman | Journal of The Electrochemical Society, 2020 167 037570 | Journal Article | 2020 |
Mode Switching with Waveguide-Coupled Plasmonic Nanogratings | T. Palinski, B. Vyhnalek, G. Hunter, A. Tadimety, J. Zhang | IEEE Journal of Selected Topics in Quantum Electronics | Journal Article | 2020 |
Development of 700 C Capable Chemical Sensors for Engine Emissions Testing and Embedded Instrumentation Applications | Darby B. Makel, Susana Carranza, Gary W. Hunter and Jennifer C. Xu | 66th JANNAF Propulsion Meeting, Dayton OH, June 3-7, 2019 | Presentation | 2019 |
Smart Sensor Platforms and Systems for Aerospace Applications | Gary W. Hunter, Darby B. Makel, and Jennifer C. Xu | 17th International Meeting on Chemical Sensors, Vienna, Austria, July 2018 (Invited Talk) | Presentation | 2018 |
Hydrogen Sensing in Titan’s Atmosphere: Motivations and Techniques | Ralph D. Lorenz, Hiroshi Imanaka, Christopher P. McKay, Darby Makel, Gary W. Hunter, Melissa P. Trainer, Robert Osiander, Andrew Mastandrea, Jason W. Barnes, Elizabeth P. Turtle | Planetary and Space Science, Volume 174, 15, Pages 1-7, 2019 | Journal Article | 2019 |
Engine Emissions Monitoring System Development for Engine Health Management | Gary W. Hunter, Susana Carranza, Darby B. Makel, Donald L. Simon, and Jennifer C. Xu | Proceedings of the JANNAF 48th Combustion December Meeting, New Port News, VA, December 2018 | Presentation | 2018 |
Portable Breath Monitoring: A New Frontier in Personalized Health Care | Gary W. Hunter, Raed A. Dweik, Darby B. Makel, Claude C. Grigsby, Ryan S. Mayes,and Cristina E. Davis | Interface Magazine, Volume 25, Issue 4, Winter 2016 | Article | 2016 |
Aircraft Ground Demonstration of Engine Emissions Monitoring System Based on a Gas Microsensor Array | Gary W. Hunter; Donald L. Simon; Jennifer C. Xu; Azlin Biaggi-Labiosa; Susana Carranza; and Darby Makel | AIAA-2014-3925, 50th AIAA/ASME/SAE/ASEE Joint Propulsion Conference, July 28 to 30, 2014, Cleveland, OH | Presentation | 2014 |
Design, fabrication, and testing of MEMS-based miniaturized potentiometric nitric oxide sensors | C.W. Chang, G. Maduraiveeran, J.C. Xu, G.W. Hunter, and P.K. Dutta | Sensors and Actuators B: Chemical, Volume 204, 1 December 2014, Pages 183-189 | Journal Article | 2014 |
A Concept of Operations for An Integrated Vehicle Health Assurance System | Gary W. Hunter, Richard W. Ross, David E. Berger, John D. Lekki, Robert W. Mah, Daniel F. Perey, Stefan R. Schuet, Donald L. Simon, Stephen W. Smith and Richard W. Ross | NASA/TM 2013 217825, February, 2013 | NASA TM | 2013 |
Nanostructured Material Sensor Processing Using Microfabrication Techniques | G. W. Hunter, R. L. Vander Wal, L. J. Evans, J.C. Xu, G. M. Berger, M. J. Kullis, and A. M. Biaggi-Labiosa | Sensor Review, Vol. 32 Issue 2, pg. 106-117, 2012 | Journal Article | 2012 |
Development of High Temperature SiC Based Hydrogen/Hydrocarbon Sensors with Bond Pads for Packaging | Jennifer C. Xu, Gary. W. Hunter, L-Y. Chen, Azlin M. Biaggi-Labiosa, Benjamin J. Ward, Dorothy Lukco, Jose M. Gonzalez III, Peter S. Lampard, Michael A. Artale, and Christopher L. Hampton | Materials Science Forum, Vol. 717-720, pg. 1191, May 2012 | Journal Article | 2012 |
Smart Sensor Systems | G. W. Hunter, J. R. Stetter, P. J. Hesketh, and C. C. Liu | Interface, Vol. 19, No. 4, Winter 2011, pp. 29-33 | Article | 2011 |
Smart Sensor Systems for Spacecraft Fire Detection and Air Quality Monitoring | G. W. Hunter, J. C. Xu, A. M. Biaggi-Labiosa, B. Ward, P. Dutta, and C. C. Liu | 40th International Conference On Environmental Systems, AIAA: Portland, Oregon, 2011; Vol. AIAA 2011-5021 | Conference Proceedings | 2011 |
Key Patents
Discover More Topics From NASA
Sensors and electronics research at NASA's Glenn Research Center
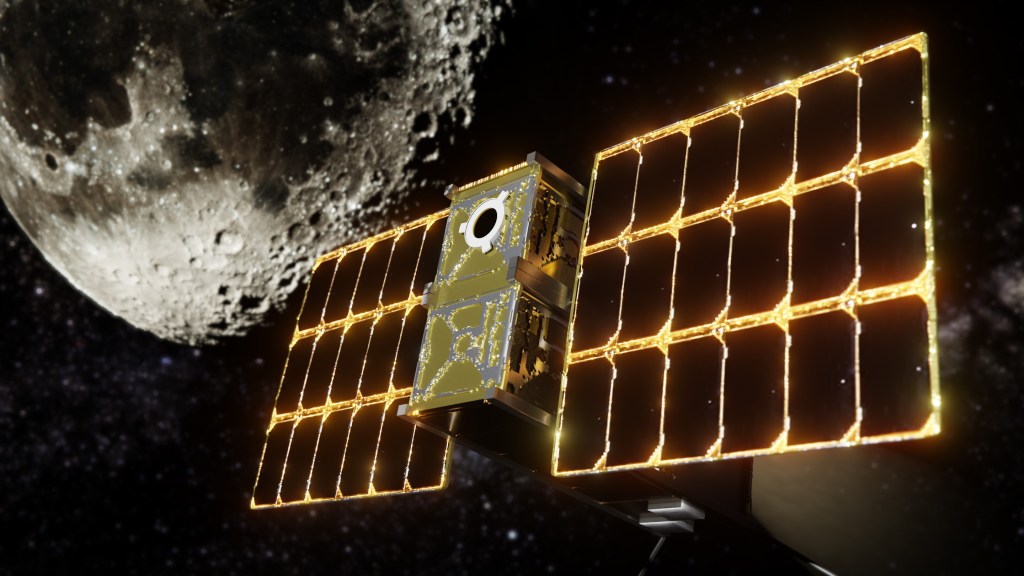
Advanced Space Radiation Detectors
NASA is developing low-noise, robust, compact radiation detectors to provide improved data on space radiation.
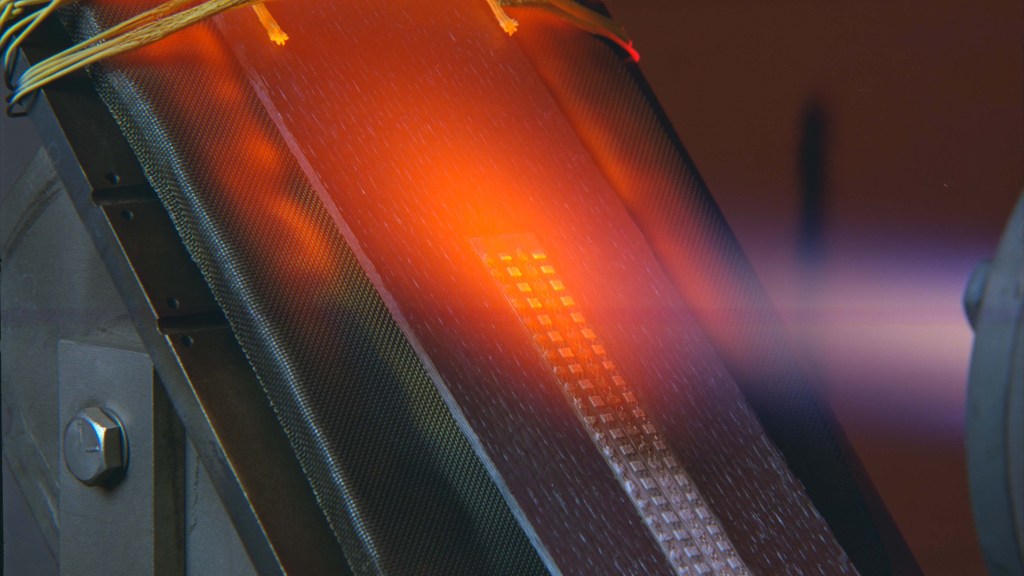
Physical Sensors
NASA is developing innovative harsh environment physical sensing and instrumentation technologies to advance next-generation space exploration, science, and aeronautics research.