From Earth orbit to the Moon and Mars, explore the world of human spaceflight with NASA each week on the official podcast of the Johnson Space Center in Houston, Texas. Listen to in-depth conversations with the astronauts, scientists and engineers who make it possible.
On episode 312, principal investigator Mark Weislogel discusses science being sent to the station aboard the SpaceX CRS-29 cargo resupply mission to improve plant growth in space. This episode was recorded on October 12, 2023.
Transcript
Host (Gary Jordan): Houston, we have a podcast. Welcome to the official podcast of the NASA Johnson Space Center, Episode 312, “Plant Water Management.” I’m Gary Jordan, I’ll be your host today. On this podcast, we bring in the experts, scientists, engineers, and astronauts, all to let you know what’s going on in the world of human spaceflight and more. We know that plants can grow in space. This is a very important capability to understand, particularly for missions into deep space, to have the capability to supplement prepackaged meals with some fresh nutrients. Now that we understand that we can grow plants, scientists are looking into ways to make that growth more efficient. One thing we’ve discovered by growing plants in space is that roots grow differently. Not the typical “up is the sky and down is the soil in space.” The roots of plants, in particular, can grow in odd ways. So one thing scientists are looking at is ensuring that those roots receive adequate hydration and aeration from germination through harvest.
Scientists are investigating a hydroponic method that may help plants grow better in space aboard the International Space Station. It’s an interesting solution, and funny enough, does not use real plants as part of the experiment design. Aboard the SpaceX CRS-29 cargo resupply mission to the station is the fifth and sixth installments of this experiment. To help describe what it’s all about is the experiment’s principal investigator who has been with the project since its start in 2019, Mark Weislogel, PhD, chatting with us from IRPI LLC as a principal scientist in Oregon. Alright, let’s get started.
[Music]
Host: Mark Weislogel, thank you so much for coming on Houston We Have a Podcast today. So good to have you.
Mark Weislogel: Great to be here.
Host: Hey, we are going to be talking about this fantastic experiment, Plant Water Management, that has been going on to station for quite some time. It keeps evolving. It’s such an interesting way to explore this phenomenon of what happens to plants in space and you know, there’s a lot to it. I’m going to have you describe it way better than I can. But I wanted to start, Mark—I usually do this with the guests whenever we start, is we begin with just your journey and just understanding how you got to where you are today, what got you interested in plant biology, and eventually working your way to doing experiments in space. I wonder where this all started. If you could take us through a little journey of really how your passion for what you do was sparked maybe in your childhood and the path that got you to where you are today.
Mark Weislogel: Okay. It’s pretty unique how this has come together. I know nothing about plants other than what I’ve learned in the last few years. So I started out my career in graduate school when I saw some NASA presentation of what liquids do in space, and I saw bubbles—gigantic bubbles—and gigantic drops. The drops weren’t falling, the bubbles weren’t rising. And I just thought, that’s another world. And it was a beautiful one and a strange one. And I thought that was neat. I wanted to do that. So I got a job actually at NASA for the first 10 years of my life. And then got a PhD and continued to work in this area doing drop tower experiments, experiments, and aircraft and spacecraft, all on liquids. So just cryogenic storage tanks, liquid fuels, liquid coolants, water processing, that kind of stuff.
Nothing to do with plants. But the merger took place when the plant folks, you know, they got to develop watering systems, hopefully someday automated watering systems and things. And then all of a sudden, it’s not so much how the plant behaves, but it’s how the liquid behaves, how the liquid can be delivered to the system. And there are all kinds of problems. All kinds of bubble problems, all kinds of droplet ejection problems, all kinds of watering problems. And so it’s easy to kill a plant by overwatering. It’s easy to kill it by underwatering it. And when you don’t know where gravity is or you can’t control where the liquid is, you’re in a lot of hurt. And so plants have done pretty well in space because they’ve been tended by crews, tended by astronauts, and they’re doing better and better all the time. And they have some great crops coming out, but it’s not always because we understand how the liquids are performing. And that’s how we got involved.
Mark Weislogel: So NASA continued to do low gravity fluidic investigations in space over the decades. And what was learned there is now really being able to deliver real solutions. And so now they’re being applied to this plant watering thing. And that’s where the collision took place. Now I’m on a fast track to learn about how plants and plant roots behave in space as well as try to deliver solutions that kind of like exploits with the liquid properties like you can’t do on the ground. I can describe those as we go.
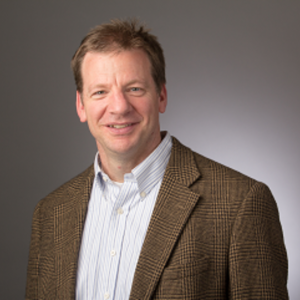
Host: Yeah. That’s perfect. And then I think that’s a really important distinction here, is your expertise, your passion really was sparked with not necessarily plants, but with water and its behavior in the microgravity environment. And this is a very unique experiment because, of course, plants need water. And that interaction in space is very unique. I want that bubble, though. That’s that’s such a unique thing to see something in space, to see an image, to see how water is behaving in space and then say, “Yeah verily, that’s what I want to dedicate my career to.” Was it really like an instant like that, like a moment where you saw some fascinating visuals of water and you say, “I want to know how that works.” Is that really what happened?
Mark Weislogel: That’s really what happened. There’s a researcher at NASA’s Glenn Research Center named John McQuillen. He was flying these low-gravity parabolas in the upper atmosphere in a Learjet. And when I saw that happen, I just thought that was so cool. My career objective became the job description of that. And I wound up actually being his office mate for a decade. So it was a really fortunate circumstance.
Host: Oh, wow. Yeah. The person that inspired you, you got to work so closely with. What were some of the things that you were working on together to better understand how liquids behave?
Mark Weislogel: Okay. There’s something called two-phase flow. It’s where you have a flow of a gas and a liquid. On Earth, no problem. The liquid is at the bottom, the gas is at the top, and they flow just fine, right? Like a lake. But take away gravity and now the lake gets all messed up, you know, it wicks away. And, you know, the giant bubbles form and droplets emerge. And when bubbles burst, they send a shower of droplets all over the place, enormous droplets. And then you wake up the next day and the liquid isn’t anywhere where you put it. How come? Because there’s been a thruster firing on the space station, or a docking, or an astronaut kicked off the experiment or anything like that. Or a change in wetting conditions. You know, all of a sudden some biofilm develops and all the liquid is sucked away. So now where you thought liquid was to keep the plant watered, it is not. Where you thought the gases should be to keep the leaves and stem dry, it is not. And so that is a problem.
We have been able to get along, but to develop the systems that have to be suitable, you know, for automated plant habitats or untended plant habitats orbiting the Moon or on the way to Mars without crews and things like that, or to try to do our best not to have the crew working on keeping the plants alive all the time. These are the situations where we need to have a very good control over what the liquids are doing so that they can grow.
Host: Right.
Mark Weislogel: Yeah.
Host: Yeah. Yeah. You want to, it’s in a sense, I guess, control. You want to have more control over what’s happening with the liquids because there’s all these factors and there’s these things that—
Mark Weislogel: Right. And you want passive control. How it works on Earth is you have a watering can, you just dump it on the plant and you let it do its thing. The water wicks into the soil, it provides the right balance of nutrients and aeration and liquid, you know, and that kind of thing to the plant. And the water level recedes as liquid is drawn into the plant. That isn’t going to happen in space. So how do we build into the watering system more and more passive mechanisms such that we mimic what happens on the ground? Mimic at least, but not kill. I mean, because in low G, it’s easy to have watering systems that wick to the plant and surround it with deoxygenated water, and the plant goes hypoxic and gets black mold, or just the leaves are bathed in liquid. So there’s lots of issues.
Host: Oh, okay. So it’s like you’re saying the plant can just hang in water. The water is trapped around its roots, and it almost, in a sense, suffocates the plant.
Mark Weislogel: Exactly.
Host: Okay. So you need—
Mark Weislogel: And so our thing is to replace the role of gravity with the role of these other weaker forces that don’t manifest themselves so significantly on Earth. Like the surface tension of the liquid, the wetting conditions of the liquid on the container and the plant, and like the shape of the container. It’s like the shape of the planter is probably the biggest thing in space, and it looks very different than it does on Earth.
Host: Okay. Very interesting. If you were to—you know, we’re, we’re in an audio medium here, so it might be a little difficult—if you were to describe the differences as best you can on how a plant is normally shaped on Earth, and those subtle changes that make things, you know, as difficult as you’re saying with understanding this phenomenon, what’s happening to the plant as it’s growing in space?
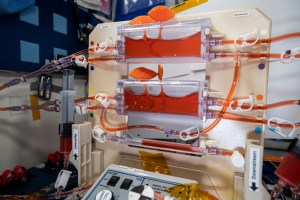
Mark Weislogel: Okay. That is actually our unknown. So some plants grow in the direction of light. Most of them do. Some grow in a direction of gravity vector and all kinds of things like that. Well, to us, it’s the shape of the container that provides the liquid in a certain way, such that the plant is getting the right aerated liquid, and it’s covering the roots and not the stem and the leaves and burying the plant and liquid. For instance, we’re looking at a hydroponic method. You picture hydroponics on Earth where you have this liquid flowing in this channel where gravity keeps the liquid at the base, and the plant grows normally and everything’s fine. But in low G, where’s the liquid going? Is it billowing up around the plant stem? Is it overflowing? Is it ingesting gas? It’s a mess. It possibly is a mess.
So we’re designing special containers that wick the liquid along in the direction of the flow. It is hard to do without a picture or two. Basically, the liquid is flowing down the channel, not necessarily due to gravity and the liquid being like that, but it’s surface tension and wetting forces and the shape of the channel now. So we do our best. Now we’ve replaced gravity. We have those other things. We have the shape of the channel, the wetting forces and surface tension to do what gravity does on Earth. Great. The thing is, what is the plant going to do? And different plants will do different things. And what I mostly mean is how do the plant roots respond to all of those forces to that flow situation in a low gravity environment? You know, do they frizz out? Do they form a braid and go downstream like they do on Earth? You know, where do they go? And so that’s why the testing in low gravity and right now we’re using synthetic roots and stems and plants, but eventually we hope to have full plants in there to add that final uncertainty of how the plants respond.
And we know different plants respond differently. And we’re working with plants physiologists at NASA, especially at Kennedy Space Center. And those folks have a wealth of information about that, but that still remains the unknown. So we can solve the liquid problem too, or we think we can, but put it in a plant, put it in low gravity, what happens to the roots? And how does that whole thing work out? That’s yet to know.
Host: Okay. Yeah. And this is why. So what you’re describing here is we’re getting right into Plant Water Management, the investigation here. When you describe this method, I wonder because one thing that’s interesting about this experiment is its evolution. We have Plant Water Management and coming up on a cargo resupply mission is 5 and 6. But there were previous variations of this and I wonder what those looked like when you were trying to come up with different solutions to understand how things are reacting. Are you evolving the shape of the containers and the mechanism of the hydroponics? Are things evolving to see how it interacts and you just change the approach of the water delivery of the water management? Or are you repeating a similar design from Plant Water Management experiment to experiment?
Mark Weislogel: Yeah, I think you best captured it in the evolving nature of the experiment. These are a combination of scientific experiments, but also technology demonstrations or engineering development kind of work. And so we’ve seen this. The first experiments were done because, hey, is this possible? And there’s a lot of, no, it’s not, and it’s unsafe. These are open channels. A hydroponics channel is kind of an open channel, and you have an open channel in the space station, you know, hey, liquid’s going to get all over the place, and you have droplets and stuff like that. It leaks and it’s a mess. I mean, grow lights in the basement of a house, you know, it’s a mess down there. There’s soil all over the place and water all over the place. You know, it’s a mess.
Well, you can’t have that in space. Well, we set out to prove that sure you can. And so the first couple of experiments were to demonstrate, yes, we can control the liquid, we can contain it safely, it can be bumped, you can have all kinds of perturbations to it. The liquid will remain in place. And then we do a set series of experiments there with fake plants that are wicking liquid and evaporating liquid up. So it looks just like evapotranspiration. It looks just like what plants do. But we model it mathematically. This is the set flow rate. This is the evaporation rate, this is the uptake rate here. Hey, well, what do you know it worked? The next one, then develop the systems preparation for passive delivery of liquid for reservoirs that continually feed liquid in as the plant evaporates the water out of the channel. So those things are being developed.
Then this next round is a pretty highly developed system with superhydrophobic surfaces and passive bubble management and passive bubble aeration and all this stuff that are necessary for this completely passive water delivery system for hydroponics. And that’s one method, right? You can have plant media, you could water soil, or you could water, do what’s called ebb and flow. There’s all these different things. And our system kind of does many of those systems. So we can test many things. What we’re doing is we’re pushing the technology readiness level of the approach so that it can actually handle real plants with confidence.
Host: Yeah. So you’ve mentioned this a couple of times. So let’s focus on it for just a second. Is the experiment, how you’ve been evolving the design to see how these plants interact with your solutions is you’ve been using, and you mentioned this a couple of times, simulated plants. So when you were trying to come up with, okay, what is a material, what is a simulant that we can actually use that is going to mimic the behaviors of plants and roots and really give us the data that we need to come up with some confidence…How did you get to the solution that you ended up at?
Mark Weislogel: Okay, so one of the things that I mentioned that completely determines where the liquid goes when gravity is gone is geometry. So the shape of our channel is kind of like a wedge channel. It has this natural propensity to exude bubbles from it. So if you saw a flow with a bubbly flow going across, you would see these bubbles rising in the channel and bursting at the free surface. That isn’t because of gravity. That’s because of the weird shape of the channel. And the fact that these bubbles are driven away from the base of the channel by surface tension forces. So that’s kind of a bizarre thing. Put a root structure in there. A root structure now will completely change the geometry surface, a block or wicking structure and change dramatically the behavior of the liquid. So we have simulated root structures that look like just a single germinated root, a bigger one, a bigger one, and a massive one that packs the whole chamber. Like happens at the end stage of growth for a hydroponic plant.
Those changes in the plant growing up completely change how you address the liquid management problem. I know it’s kind of hard to explain without a sketch or two, but it’s just like saying you have a different container when the plant is germinating versus when it’s fully formed. And so you have to have a solution that can passively respond to those geometric changes as the plant grows. Very strange things happen. You have a single root from a germinating plant. You drain the container, the root sticks to the wall, you flood the container, it reenters the wall. It’s actually changing during the process. It might get convected downstream and clog the hole at the exit. But it can also grow upstream and clog the hole at the entrance. How does your design adapt to that?
So these are the kinds of things. And so we go to Hobby Lobby and pick a special root-like wicking structures. And then we make a stem, we make a route, and it has the same properties, the same wetting properties, the same wicking properties, and kind of goes after the same geometry. We grew a bunch of plants and these test cells on the ground. We did all this analysis counting how dense the roots need to be snipping them apart, building them, so we’ve got something that’s pretty good, and it’s just standard materials that are safe for the space station to check out these models and how the liquid behaves.
Host: Makes sense. Okay. Hey, let’s get into kind of better understanding what some of the issues are when it comes to this. Kind of trying to bring it back as high level as we can to really help us digest just what are the issues that we’re trying to solve. And one of them that you mentioned a couple of times is this is a passive system, right? So when you try to try to capture what a passive system is and why that’s important to design, I think you mentioned one of the things was for the plant experiments that have been in space a couple of times required a lot of crew time and watering and things. So what’s important about investigating a passive design?
Mark Weislogel: Well, there’s a bunch of reasons for plants in space. And one of them is the crew. Some of the crews especially enjoy interacting with them. It hearkens back to Earth and stuff like that. But there’s always this push for automated systems. The crew time is so valuable, being used for other things is competing. It competes for that time. So you could imagine a satellite that’s been sent to orbit around the Moon without any crew on it at all. And by the time the crew arrives, you’d like to have this crop ready for harvesting or whatever, whatever using the plants for, you can use them for air revitalization as well as food and, you know, just a whole bunch of stuff. Well on Earth, we’re just really used to the passive role that gravity plays. For hydroponics especially, liquid is injected upstream, gravity drags it across the base of the channel, and then it falls into a chamber. It bubbles in, and aerates naturally, and that cycle repeats that we’re so used to that. Well, engineers would love to have the equivalent of that in space. So that’s a passive system.
There’s a pump that delivers liquid, but it’s gravity that makes that liquid flow and gives us their aeration. Trickling water into a bath of liquid is producing all these bubbles that aerate the liquid, but gravity is naturally separating all those bubbles. The bubbles rise to the top, the liquid falls to the bottom. And voila, we can still suck up li a hundred percent liquid and send it back to the channel. Not so in space, you did that in space, you would make a frothy foam kind of thing, and you would never be able to access liquid, and you’d be ejecting bubbles upstream and, you know, it’s a mess.
So how to do that? So what we’re aiming for is we’re using geometry, the geometry of the conduit, the geometry of the valves and fittings, the geometry of the primary channel, hydroponic channel to do that passive separation for us. And those technologies have been developed, you know, in part for liquid fuels on rockets and liquid coolants and radiators, space radiators and stuff like that. But they haven’t been applied in mass to plant watering. And it’s time, it’s possible now. And so that’s kind of what we’re doing. We’re applying that technology to these problems, trying to solve all this so that the automated system can go up, flip a switch, prime the system, germinate the plant, and provide the right amount of liquid in the right way, just like they do on Earth but in an automated fashion from the ground.
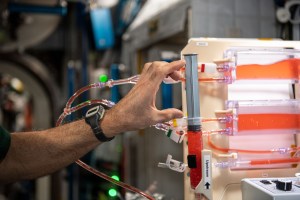
Host: And that’s the trick. Where gravity is helping you out with the systems on the ground, you are looking for those forces. And it sounds like surface tension is one of those things that play a really big role in the design where gravity is a predominant force in designing systems here on Earth, not so much in space. So when you look at something that has a passive design, surface tension is one of those driving forces. And you mentioned some interesting things and using hydrophobic surfaces, you mentioned a lot of pieces that are in the design that help you to manage that water. I wonder, if I’m reading that correctly, if surface tension is one of the significant forces that help you with the design, or if there’s maybe other forces that I haven’t really addressed or that I’m missing.
Mark Weislogel: No, surface tension is the primary force. But surface tension has no means of doing any action at all unless there is some sort of favorable wetting situation. So it is surface tension combined with wetting, and then in a more complex, but equally important role is the geometry of the system. If you just had a cube, for instance, you would have no control. But if you have a tapering pyramidal kind of thing, you do. So it’s like surface tension, wetting, and container geometry that make this happen. Now, on Earth, you stick a tube in water and it wicks up a little bit of distance and then stops. That’s cause gravity is limiting that flow. But in zero gravity it’ll wick forever. So you can either get burned by something like that happening, or you can exploit that. And we’re trying to do the latter.
Host: Exploit. I see. Okay. So, when it comes to a couple of things that you mentioned, you mentioned this a little earlier when it comes to, you know, how to water a plant in space, right? There was this phenomenon that the water, because of surface tension, would stick to the roots and almost, you know, suffocate them or drown them. You also mentioned dealing with bubbles and coming up with a passive way to move bubbles, almost like you would experience them on Earth. So let’s focus on the bubbles here for a second on how that plays a role in making sure that—because I think one of the things that’s part of the experiment design here is you’re dealing with hydration and aeration. So obviously controlling and managing those bubbles has got to be a significant part of successfully managing how much water a plant is getting, right? So where do bubbles come into your design here?
Mark Weislogel: Okay, so a typical hydroponic channel looks like the gutter of a house, almost. It has a rectangular base, a flat base doesn’t. That’s fine because bubbles that are injected into that channel rise to the surface. They leave. That’s okay. In space, not so. If you had a rectangular channel and you had bubbles, they would just ride through the liquid, build up in some location, and perhaps displace liquid out of the channel and it would spread all over the place and not be good. Not only that, but a large bubble could form around the root zone and then suffocate, you know, dry out basically the roots. So that’s not favorable either. But now, we use a channel that in its most simple way is like a triangular base. It’s like a wedge. And so bubbles at the base of that are actually driven away by surface tension out of the wedge. So there is a natural tendency for bubbles to “rise” even in low gravity. And so they can rise right out of the liquid and leave before encountering the root structure or even within the root structure. So I don’t know if that is clear enough there, but there is a mechanism to remove bubbles by just the geometry of the channel.
So that’s the first breakthrough or the first application to this process. But then what? Okay. So, you wouldn’t like bubbles in the channel anyway. So that’s like a backstop. So if you inadvertently get bubbles, the channel can kind of take care of them. But we need to aerate the liquid. So we use an aeration system that produces bubbles, and then we use special devices, like these are like fluidic, capillary, fluidic devices, that then do passive separations of those bubbles upstream. So it’s like a bubble filter upstream. So we actually have other devices in line. And this is what our experiments are out to demonstrate, is that you can handle. So if you produce bubbles on purpose by aeration or by accident by ingestion, you can get rid of them. Bubbles can—there are many things that could be said about them. I mean, as I said earlier, I’m fascinated by them cause, in low gravity, they move differently. They’re gigantic. When they burst, they erupt. I mean, when they burst, they form films, they shoot out droplets. When a bubble bursts on Earth, you can’t see it happen cause it’s too fast and too small. But what’s happening at burst, is there’s a wave that ricochets around the bursting bubble. It forms a geyser and shoots a jet out with a ton of little droplets, that are on Earth. And you can see videos of that kind of stuff on YouTube if you want to by high-speed video microscope cameras. Okay. But in space, those droplets are giant. So if you have bubbles bursting in a channel, you’ll eventually shoot all the liquid away out of the container, or it’ll be a mess.
Knowing those things and how that happens in the microgravity environment, that’s when we make walls out of these superhydrophobic materials that just rebound the liquid right back to where the liquid came from. So there’s a bunch of like nerdy fluidic mechanics things that are built into this plant thing to make it work. And it’s surprising how many it needs just to make it perform like a gravity one. So the plants you grow on Earth are like the plants you expect to have in space with a nearly passive system. All you have is a pump.
Host: All you have is a pump. Interesting. Okay. So, more on the bubbles here. And you talked about aeration. And I wonder, is aeration an important thing for the health of the plant? Does it also play a role in, cause you talked about introducing bubbles, in the passive design and the actual flow of the water and making sure that the water is controlled and predictable in its flow? Maybe it’s a little bit of both, but what’s important about aeration when it comes to watering plants with a hydrophobic method?
Mark Weislogel: Yeah. All plants need a degree of oxygen to the roots in order to thrive. So they need nutrient water and the right oxygenation. So you need to provide aerated water. Now, in low gravity, it’s pretty hard, unless you have aerated water delivery. Eventually, the oxygenation diffuses out of the liquid, and you have oxygen-starved water. And in low gravity, the liquid just balls up around the roots and then suffocates the plant. Not because it’s not getting nutrient in water, but because it’s not getting any oxygen. And then they suffer all the hypoxic consequences of that for the plant. So you’ve got to deliver oxygenated water. So we have a passive aeration system that’s upstream of the pump. And what as the pump is operating, is drawing in plenty of oxygen into the water stream. Unfortunately, why did you do that? You just made a bunch of bubbles. That’s disaster in space. But we’ve developed these devices that are upstream of the channel that for the bubble’s frequency and size and dense that we’re introducing, we can filter those. So then a hundred percent bubbles in, a hundred percent bubbles out before the channel. And now you have a hundred percent oxygen-saturated water, aerated water, basically for the plant. And now you’ve solved that problem. That was an absolute no-no, I’d say 10 years ago. No way. No way do that. You’re just introducing disaster. But again, because of that research NASA has been performing all this time, it’s now possible, in fact, very possible with margin, you know, to make systems work this way.
Host: Yeah. That’s amazing. The evolution and the continued science got the scientists around to saying, “Hey, maybe there’s a way we can, we can make this work.” Alright, so we’re talking aeration and I want to circle back, because you mentioned this as well, when it comes to the design of your particular experiment, we’ve been talking a lot about water and the geometry of the actual delivery mechanism and aeration. You’re right, this is tough to do without visuals, although, in our show notes, we’ll show as many visuals as we possibly can to help the listeners for those that want to go and check out how this experiment is designed. But one important thing is that, you know, you mentioned nutrients and part of the design of this particular experiment is no soil. So those nutrients aren’t coming from the tip. When you think about plants growing on the ground, you don’t just plant them in the dirt in the ground. This is soil-free. So you talk about the oxygenated water. You talk about bubbles, talk about water. How do you introduce nutrients into the hydroponic design?
Mark Weislogel: Okay. With the help of the NASA Kennedy scientists, there are several different ways to do it. The way we’re planning on doing it is to have a reservoir where the liquid flows through and it has a packed bed of particulates. And this is very commonly used on the ground too. And they have like a time release. And as liquid is moving through that bed, it slowly dissolves out that nutrient at a rate that the plant can handle. So that’s currently the passive means of doing it. The release agent there, that nutrient pellet there, is what is developed on the ground, and then that’s flown. So that’s the plan.
Host: Yeah. The water passes through these nutrients and then delivers it right to the plant. And I’m guessing that is a consumable, right? You only get so many nutrients, and then maybe you need to replace, or you need to continue to supplement or provide the nutrient packets or whatever it is.
Mark Weislogel: Exactly. Then they eventually dissolve completely away, and you just replace that cartridge.
Host: Replace the cartridge. Okay. So again, minimizing crew time, and when it comes to crew time and this design of watering plants, you’re replacing cartridges. Is it minimizing the crew’s interaction with actually watering the plant? What else do they have to do?
Mark Weislogel: Well, yeah. The crew could do a bunch of things. I mean, they could do certainly the harvesting, maybe the pruning, you know, just a checkup now and then but eventually you can imagine a camera on every plant. You know, these cameras are so tiny, you know, a camera on every plant, and it’s monitored from the ground. So your gardener’s on Earth, you know, that’s what I picture. But maybe there’s a fully automated mode. You just said it and forget it. But I can imagine that the scientist wants to watch this crop develop from the ground that, so I picture that. You can do for so affordably, so safe, so certain every plant gets its own camera. And those images are coming down either daily or video, depending on what you want.
Host: Okay. Yeah. So what about the experiment then? How are you getting the data that you need? Are there cameras on your experiment? Are there instruments to measure oxygen levels or something like that? What kind of instruments do you have?
Mark Weislogel: Yes. That’s the best part. These experiments are performed in the open lab on what’s called the MWA. The MWA is Maintenance Work Area, and it’s basically a workbench. And they lay out the plumbing and they set up a camera and they set up lighting. Then they turn on the pump and they adjust this, and they adjust that. And we get this beautiful high-def image of everything. And so one video, live video, coming down from space gives us flow rates, gives us bubble velocities, bubble sizes, bubble distributions, liquid levels, you know, it gives us everything. So it’s great. I’m just guessing maybe a dozen or so parameters we measure from a single video. Then we’re working with the crew. We’re talking to them over, we can adapt to discoveries, we can adapt to off-nominal behavior. And these experiments, do largely to the crew time that we get, have been extremely successful. They always add more science. They’re always capable of something new because you can work with it. And so that’s made all the difference.
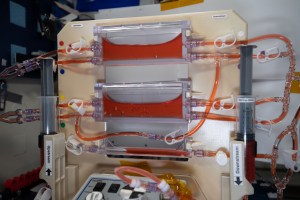
Host: Oh, okay. So it sounds like the design of the experiment is you have runs. So you set up a time where it’s not just constantly flowing. You set up a time where you want to see how it flows, and you can interact with an astronaut and have real-time communication, but you do it in a set period of time, right? Is that sort of how it works?
Mark Weislogel: Right. Usually, our thing is we set up one day and then the next day we prime the system and get going. And we’ve operated between eight to 12 hours a day for a couple of days. Then we usually change out the test cell and do it again and do it again. So that’s how it’s been.
Host: What’s the test cell? What do you change out?
Mark Weislogel: We can change out the way the test cell is plumbed, we have hydroponic channels that are in series. Then they’re in parallel. Some have access to these passive reservoirs, some access these passive bubble separators. And so there’s a bunch of different geometric things that are tested in between. And then we vary the plant root models from ones that completely pack the channels to ones that are more representative of germinating plant roots. So there’s more to test than can possibly be tested. But we have crew procedures that are developed for this optimal set. And then we can go from there. We have extra procedures that are in the appendix, basically, of the crew procedures. And if we have more time, we’d go to those. If disaster strikes, we can recover. And we’ve always been able to get more from the hardware than was planned. Mostly because of the flexibility of interacting with the crew and their capability.
Host: What a fascinating experiment to be a part of. You send something up to space with this ton of flexibility, and in an experiment run, you say, “Hey, let’s try this. Let’s try this, let’s try this.” And it could be the way the water flows, it can be the types of plants that you want to try out. And it could be plants at various stages of their lifecycle, from germination to harvest, whatever. There’s so much flexibility built into an experiment. That’s awesome.
Mark Weislogel: That’s right. And NASA doesn’t necessarily like that really. There’ll be a certain place in the crew procedures where the crew is working down through and we’re talking to them where it’s really vague because we know that we don’t know what’s ahead. So we’re trying something, but that’s new. A new demonstration. Then well, what do you know? The liquid breaks forms of film and can’t even do what we expected. So then we get this idea, “Oh, open that valve. Do this. Change the pump like this.” But NASA needs to know that that is an extremely productive time cause that’s the time where you’re really learning new stuff and you’re going, “Oh, no wonder we’ve been working in this field for decades and we still get surprised like this.” And when we get surprised, it’s always thumbs up because now it’s either, now we know how to avert disaster, or we know how to exploit new forces so that we can develop new solutions. And so now hats off, hats off to those people in the NASA cadre on the ground who still allow that kind of stuff. Because that’s discovery. It’s discovery with the crew and hardware and the research team on the ground and the cadre’s sitting there watching.
[Laughs]
Host: That is such a cool experiment design. Just rolling with the punches. Hey, that’s new. Let’s try this. And I think that probably had a lot to do with, and we talked a little about this a little earlier in our discussion here, is the evolution of the experiment. As you find new phenomena that are happening and make discoveries along the way, it better informs your design. And so leading up all the way to now, Plant Water Management 5 and 6, Mark, what are some of those phenomena that you have learned through all of this trial and error and investigating new things and finding new phenomena about what’s happening in space? What are the things that have informed the design of these upcoming Plant Water Management systems that you think will take it to the next level?
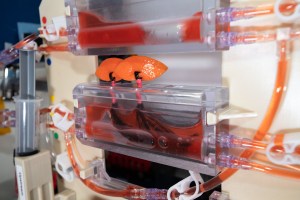
Mark Weislogel: Okay. What comes to me first is probably something that is going to be the least effective. I don’t think I can share this in a very—it’s going to come out like complete geekiness, but let’s do it.
[Laughs]
Mark Weislogel: Okay. A long time ago, it was thought that you can use capillary forces, basically surface tension, only if you got perfect wetting. So that’s like water on glass, but water on no other material. It’s like a fuel, a cryogenic fuel that’s on a metal. Those are perfect wetting systems. Or an oil or a coolant on a copper tubing. So then they thought, okay, when it’s partially wet, when it doesn’t wet perfectly like water on your windshield, that’s not perfect wetting. Those little droplets, you know, it’s not perfect wetting. You can’t do anything with that. It won’t wick and flow. It just hits there and sticks.
So I would say what we’re doing now is like, we’re exploiting the fact that you have crummy wetting. And we’re demonstrating it. So we do it again with geometry, with flow. We use inertia of the pumped liquid. We use pinning edges, we use super hydrophobic, we can do that. We have special materials that we’ve invented and are applying them in this experiment, you know, for the first time where the fluid actually just bounces off the surface cause it can’t wet it. That’s killer. Wherever you put a surface like that, it becomes the top of the surface, top of the container. Ah. I don’t know if that’s coming through, but—
Host: No, it is, it is. You’re talking about controlling, having a better understanding and controlling where the water goes. That’s really what it is, right?
Mark Weislogel: Yes. Yes. And we did not believe that before. And lots of people would say “You can’t do anything with about in a system like that.” But now we’re saying, “Oh yes, you can.” In fact, we’re showing that it’s better. Because the liquid stays put better. It’s more stable when it’s crummy wetting. And we’re doing this for plant watering, but we’re discovering this with through the plant watering experiment. And then we’re applying it to other things. We’re finding out that it’s true if you’re handling urine in space, vomit in space, diarrhea in space, saliva in space, blood in space. So it’s not like the approaches here are isolated just for Plant Water Management. It’s like you’re discovering that you can handle poorly wetting fluids, contaminated liquids like that. And there are contaminated. We’ve got water with plant debris in it. There’s biofilms in there, there’s nutrients in there, there’s hair in there. You know, it’s a mess. Fragments of roots. But we’re still learning that these methods are robust to address those things. Even exploit them. Because as the wetting properties change, the system gets better and better.
We’re also learning that there are two ways to design this. One for crappy wetting. One for good wetting. And you can actually use both methods on the same design. So the same design has both capacities. It can handle both systems. So it’s becoming more and more robust. And the more opportunities we get to demonstrate this, the more advances take place. Pretty soon NASA’s going to be handed a technological approach for this kind of stuff. And it’s going to apply not just for watering plants, it’s going to apply to, really, urine processing systems and space. A big one, right? Water recycling in space is a big one. And in that process, there are 50 challenge problems. And each one of them, you could point to a plant watering solution that, you know, it’s kind of like that. Not a hundred percent, it’s like that. But it is kind of like that.
Host: Well, it is exciting. You’re talking about broader applications, not just Plant Water Management, but just total microgravity water management. The broader applications are really important here. So, Mark, how are you and your team sharing the data then? When you come up with these solutions and you have these new understandings of how water behaves, how is the scientific community talking to each other? So yeah, maybe the person designing the next urine management system has a better understanding of how to properly channel that urine and make that system that much more efficient.
Mark Weislogel: Right. We publish our results and we present them for sure. We publish them too. This one has been such a race because it’s like “Go to space, go to space, go to space.” So this one’s been such a race that we don’t have a tremendous amount of published work on this, but we sure present a ton to NASA. We have published in conference papers. These results are being included in a book chapter. I know we have a few journal articles that include it as well. So it’s definitely being published to get the information out there. Some of it’s strange to report cause some of it doesn’t have the foundational science behind it yet. There are some, again, some very nerdy aspects of these flows that we think are decades away from being understood. But the engineering application is here and now, so it’s like, okay, we don’t understand turbulence that well. I mean, we really don’t, we don’t understand turbulence that well, but we still can design fighter jets, you know? That’s this situation. We’re decades away from truly understanding some of the aspects of this flow phenomenon. But it’s not stopping us from being able to design workable systems that can perform to engineering specifications. Yeah.
Host: Maybe it’s through working towards that, through working towards finding out how to best manage it and finding out the systems and the engineering towards it that maybe helps us understand the science that much better progressively. And you said decades, right? But maybe just continuing along the path that you’re on, Mark, with just learning and learning and coming up with designs that inform the science, the broader science.
Mark Weislogel: Right. So we come from the science background, so we know what those science challenges are and we’ve developed the special computer codes and things like that to do some of these analyses. We’ve done the mathematics oftentimes for the basis of this, but that uncertainty that I’m saying is decades away is in all of those things, but we’re getting access to space and we’re filming it. Then just like you were saying, we are developing the engineering tools then to build the next system. So we are developing a database from which to design these systems, even though we don’t necessarily know how the liquid is holding on. When you have a drop of water on a surface and it’s sitting there sticking and you tilt the surface, you tilt it up and up and up and up and pretty soon it lets go. You know, what are all the tiny little forces that are present when it lets go? We don’t necessarily know those things. We can measure them and there’s a protocol to do that, but those are the kinds of things that are in the details. They’re critical, but we can still make systems work though we don’t understand them fully.
Host: Yeah. So that’s what you’re working on now with the Plant Water Management system, the systems themselves. And of course, we’re talking about 5 and 6 and some of the ambitions you have for those. Is your approach going to be similar? And you’re starting to get scientists around to that method that we were talking about a little earlier, Mark that was built into an experiment run is flexibility. Do you think the scientific community is coming around to understanding the benefits of building that into the experiment runs? Can we expect to see that for 5 and 6?
Mark Weislogel: I would say if you change it to instead of say scientific community, you say engineering community, I’d say yes. Because now I think people, at the beginning, in is as little as two years ago, I would get a lot of resistance when I would present this material either at a conference or even in a paper during the review process, because you know, is it real? I mean, I don’t know if you remember, but we were actually part of that coffee cup design that NASA demonstrated years ago.
Host: Oh, yeah. With Don Pettit.
Mark Weislogel: Yeah, with Don Pettit. And that coffee cup kind of exploits some of the things that are applied here. So the coffee cup actually proceeded this showing that you can make surface tension geometry and wetting replace the role of gravity. And astronauts were drinking it from that cup naturally as if there was gravity, but there wasn’t. How come that was working? The reason that was working is the same way this is, and I would say this is a follow on to the coffee cup in a way. But at the beginning, even NASA safety didn’t agree you could do this and you shouldn’t be able to trust that the liquid will stay in the cup. So that wasn’t very long ago. And that there’s still some of that pressure on these kind of designs. But that’s going away because now that these are being demonstrated and people from safety engineers to plant physiologists are saying, “No, this is how you can do it and this is the way you should do it. And it is safe.”
I mean, when that first coffee cup was drunk from, it was a scalding hot liquid in an open container in the cupola. Remember when Samantha Cristoforetti did that? It was incredible. It was a no, no, no. It was a trifecta. Rejected, rejected, rejected. But it was a delight and everybody on the ground was laughing and enjoying it. How come? Because it’s legit. I mean, surface tension, wetting, and geometry provide a level of control and you can exploit it. And this is really doing that. We’re really exploiting it. We’re really showing that plant watering can be done in a way that you would expect the plant on Earth, on an Earth level of expectation. Instead of where’s the liquid? Or next time you turn on the camera, you find out the entire plant is buried in a blob of water, you know, or that kind of thing. Or you turn on the camera the next day and where’d the water go? Oh, wicked over to this other reservoir where, no, that’s not where we want it. And we have to monkey around with it. Okay. Let’s get the crew back over here and help us out. You know, that kind of thing.
Host: Yeah, fascinating. So Mark, what are your ambitions then for 5 and 6? Do you hope to mature the design and maybe we’ll start seeing some real plants in the next iterations of Plant Water Management? What are your ambitions for the upcoming experiments?
Mark Weislogel: Yeah. 5 and 6 are the, I would think the cherry so far. They demonstrate pretty much a full engineering system but just for two test chambers. The chambers are bigger. You can grow giant plants in these if you want to. We have on Earth, you know, these are, these are large plants, you know, two feet tall, giant big canopies from a small wedge container with tons of roots in them. So we’re going from a germinated root model to a fully packed model, we’re testing with full aeration, bubble separation, water traps, bubble migrated systems, superhydrophobic surface to rebound liquid water. It’s got pretty much it all. So much so and with confidence that NASA is actually pursuing making farms of these channels. So they’re special microgravity channels, but they perform on Earth too, which gives us this omnigravitational approach. It will work in a gravitational environment on Earth, on the Moon, on Mars. The cool thing is it works in microgravity and that’s the most challenging one. So your plant system could target all of those environments and not be that concern. This whole 5 and 6 is after that demonstration. And I think NASA’s recognized that. And so we’re providing a channel farm to NASA Kennedy to work on this next generation, which might actually use ultimately, say the Veggie, which is on space station and grow real plants from germination full to harvest. And that is the real objective because there still is an unknown.
That unknown is how does this type of plant in microgravity, in that channel respond? What is its root zone? Is its root zone some spastic thing? Is it highly ordered? Is there enough convection in the flow to make it just like Earth? That would be incredible. So if we have enough convection in there to generate a plant root structure, which is just like Earth, then now you have a model in space which mimics the model on the ground and now you can go to town with your architecture of your plant growth facility. How you order the plants, how you order them with lighting, how you order them from the germination step when they’re all condensed, and then pull them apart, spread them apart, and grow this large plant habitat. So that would be a certainly exciting trajectory if that’s what NASA decides to do if they’re convinced. And that’s what we need to show.
Host: Yeah, the broader applications and just making plant growth in space that much better. Mark, I wonder if there are any Earth-bound applications as well. I know you mentioned the design of Earth-bound systems heavily relies on gravity. And of course, a lot of what we’re talking about here is we don’t have gravity, let’s figure out how to make it like it is on Earth. But I wonder if just better understanding the flow of water and these phenomena that are happening in space if that in any way can maybe translate to something to make things better here on Earth.
Mark Weislogel: I don’t know. I know that the system on Earth is so much simplified by gravity doing everything for you. It does all the separations for you. It does the aeration, it does everything. But now that would be the plant physiologist who would make that connection. And they have had tremendous input on the direction of this work. And so the hydroponic system is interesting because the plant roots remain super supple, and so they’re 30% more productive in terms of the plant above the surface. So hydroponics is a unique way, a very productive way to water plants. Now, if there’s something unique about this geometry and how it makes the root zone a certain thing, there might be something there. But that’s really out of my understanding cause now you’re back to the plant physiology and those guys are all over the place, you know, in what’s actually going on with the plant. They love plants, but they know the science behind them, that’s not us.
Host: Right? But you said you published the results. So maybe there’s someone out there with a crazy idea that that may take just a fraction of the total application of everything you’ve worked on and apply it to something. You know, I think that’s the beautiful thing about science and these investigations is there may be some questions that we haven’t even asked yet. And there are answers out there and based on everything that we’re doing, and it’s really just a lovely thing. Mark, I want to kind of end this by circling back to the beginning when you talked about your passion for understanding how water works in space and seeing that bubble, and then light bulb went off and you started pursuing this. Now you’ve gone down this path of working with Plant Water Management for quite some time. I was excited as you were telling me about those videos that you were seeing and the runs of the experiment and kind of going with the flow and seeing something and saying, “Wow, let’s go down that path.” I just wonder, you reflect on your career and the work you’ve done, and I wonder how it sits with you now as we’ve been talking about this on all the work that you’ve put into better understanding water in space and just reflecting on your career and your path that got you to where you are and where we’re going. How that sits with you at the end of this conversation.
Mark Weislogel: I bet a lot of your guests are kind of people who just really love their jobs. I mean, working in space, working with NASA is an incredible experience. I’ve never gotten used to it. I’ve not gotten used to any of the space encounter, or not any of the crews, any of the astronauts, can’t get used to this. It always feels like this. In this project, when I’m seeing so many different elements come together, again, with drops, films, bubbles, jets, bridges, droplets, Cecil droplets, pen droplets, I mean, all this stuff, it’s a total nerd out situation. I can get pretty darn annoying. Really, I can get pretty annoying because it is like this. To me, again, if you look at a sequence of videos of things that people have done in space, we have several of these things. They come off in a presentation. It is just wonderful. It’s wonderful to see phenomenon that is unearthly, like a large droplet. We’ve done like pinging pong paddles with water in space. And we had these crew just test out this non-wetting surface for us. They were hitting back and forth a one-liter size droplet with pinging pong paddles. I sit there and just stare at that, and they’re just dinking around. But that kind of phenomenon is a beautiful thing to watch. The fact that it’s important, it’s necessary, it happens. I mean, especially for these large-scale missions to the Moon, fuel depots where the liquid is so big, it has its own gravitational force, you know, fuel depots and that are headed to Mars and, and all this stuff. There are some real challenges for exploration just in liquids.
You know, the areas are vast combustion and material science and the human anatomy, and food. There are so many things that we need to understand well in order to go to explore safely. But just the micro thing of liquids in space, it’s called fluidic physics, and NASA pursues this area. There’s just so many avenues. It has to do with hygiene, you know, showers in space, toilets in space, all kinds of water processing, coolants, liquid fuels, you know, propel propellants, body saliva in tear ducts and in your mouth and in your gut. I mean, all of these things. And they’re all connected by the fact that, hey when gravity’s gone, what happens? Oh, surface tension, wetting, and geometry. Whether it’s a biological system on a microscope slide or whether it’s a condensing heat exchanger surface in an astronaut backpack. It’s all liquids in space. And it oftentimes behaves completely unexpectedly. And I don’t know why that turns me on, but it does, and I love that I’m around others that are like that. It makes it pretty exciting to do this work. And that’s why I say get pretty annoying. I mean, to be around a person that’s like that has got to be pretty annoying.
Host: I don’t know. It could be energizing too, in a sense. You know, someone who’s so passionate and so excited as they’re going through an experiment saying, “What’s that? I wonder what that is. Let’s investigate that.” And just the energy I feel, at least for me in this conversation, Mark, has been contagious. It’s been an absolute pleasure to talk with you and get to feed off of a little bit of that energy and passion for fluids in space and how liquid behaves. It’s just such a fascinating thing. And like you said, we talked Plant Water Management today, but the applications are so broad. So, Mark, it’s been so cool to talk with you. Thank you so much for coming on Houston We Have a Podcast and talking about these great experiments and all we have learned and have yet to learn in the world of fluids in space. So thank you for coming on.
Mark Weislogel: Oh, you’re welcome. I enjoyed it.
[Music]
Host: Hey, thanks for sticking around. Mark brought some contagious energy to the conversation, and I thought it was absolutely fascinating. Definitely learned a lot about liquids in space, and I hope you did too. You could check out nasa.gov for the latest that’s going on aboard the International Space Station in particular. Make sure you navigate there because this is just one of many experiments that are being conducted every day aboard the orbiting laboratory. We are one of many NASA podcasts across the whole agency, and you can check them all out at nasa.gov/podcasts. That’s where you can find us and listen to any of our episodes in no particular order on social media. We’re on the Johnson Space Center, pages of Facebook, X, and Instagram. Use #AskNASA on your favorite platform to submit an idea for the show or maybe ask a question and make sure to mention it’s for us at Houston We Have a Podcast. This episode was recorded on October 12, 2023. Thanks to Will Flato, Dane Turner, Abby Graf, Jaden Jennings, Dominique Crespo, Destiny Doran, Melanie White Lyons, and Keara Mangan. And of course, thanks again to Mark Weislogel for taking the time to come on the show. Give us a rating and feedback on whatever platform you’re listening to us on and tell us what you think of our podcast. We’ll be back next week.