May 2024
Interesting Fact: Behavior of Liquids in Space
Here on Earth, we all live in a state of gravity. Not only us, but everything around us, including water, is being pulled towards the center of the planet by gravity. True, it is nice that our dogs don’t float off into space, but when a child drops their ice cream (which is full of water, by the way) they don’t have to know about gravity to be upset. But, if you go far enough out in space, for instance to the International Space Station, gravity becomes negligible, and the laws of physics act differently than here on Earth. Just how might water act in a place of zero gravity? The photograph below of the water drop and air bubble gives you a good idea of how differently water behaves when the effects of gravity are counteracted
This unique picture shows not only a water drop but also an air bubble inside of the water drop. Notice they both behave the same….according to the laws of physics in space. They both form spheres. This makes sense, as without gravity to tug downward, the forces governing the objects are all the same. So, the water drop (and air bubble) form themselves so they occupy a shape having the least amount of surface area, which is a sphere. On Earth, gravity distorts the shape, but not in space.
Consider what would happen on Earth: The air bubble, lighter than water, would race upward to burst through the surface of the droplet. In space, the air bubble doesn’t rise because it is no lighter than the water around it—there’s no buoyancy. The droplet doesn’t fall from the leaf because there’s no force to pull it off. It’s stuck there by molecular adhesion.
Sticky water. No buoyancy. These are some of the factors spacefarers must take into account when they plan their space gardens. If water is sprayed onto the base of the plant will it trickle down to the roots? More likely it will stick to the stem or adhere to the material in which the plant grows. As humans spend more time and go farther out in space in the future, the physics of “space water” will need to be well understood.
Boiling is the conversion of a liquid into vapor. Boiling is used every day to cook food; generate steam to heat buildings or turn turbines; cool electrical generators; purify water; and help convert crude oil into gasoline.
A pot of boiling water is something most of us can picture in our heads. Think about the role gravity plays in this everyday phenomenon. As the water heats, gravity causes the hotter regions of liquid to rise–a process known as “convection”–which distributes heat throughout the water. Once the water heats to the boiling point, the gravity-induced buoyant force sends the bubbles upwards, producing a “rolling boil.”
But this familiar picture changes drastically in a microgravity environment. Gravity-driven convection and buoyancy are non-existent, and without their dominating influence, scientists grow closer to understanding the fundamentals of boiling.
The Boiling eXperiment Facility — Microheater Array Boiling Experiment, or BXF MABE — contains 96 individually controlled microheaters provided both location and time-dependent information to gain better insight into the boiling process. The experiment, which launched on STS-133 in 2011, was a collaboration between the University of Maryland in College Park and NASA’s Glenn Research Center in Cleveland, Ohio. It was one of two experiments in the station’s new boiling facility.
“Boiling is heavily influenced by gravity,” said Jungho Kim, principal investigator for the experiment at the University of Maryland. “The vapor bubble is less dense than its liquid; consequently, it wants to rise through the liquid. By reducing the effect of gravity, it becomes possible to examine other variables, such as how hot the heater is, how warm or cold the average liquid temperature is, and the effect of pressure and surface tension.”
Given our dependence on boiling liquids in food and drink preparation, it is easy to imagine why scientists are interested in studying boiling phenomena. But, beyond the kitchen, boiling is used in many engineering systems as an effective means of transferring heat. Excess heat can be dispelled through the vapor bubbles moving through a boiling liquid. Although boiling figures prominently in the mechanics of heating/cooling systems, power plants, and engines, a better understanding of this phenomenon could lead to more efficient and effective applications.
Pool boiling experiments conducted onboard an orbiting space shuttle provided scientists the first glimpse of boiling in space. In gravity, vapor bubbles form on the heater surface of the liquid and, due to buoyancy, quickly rise to the top. In microgravity, without buoyancy, the bubbles remain attached to the heater surface and continue to grow. As these bubbles grow, the liquid is no longer in contact with the heater surface to cool it down and therefore, the liquid is useless as a heat transfer device. This situation can lead to “dryout” which is the suspected cause of the infamous Chernobyl disaster.
James Bond might be the first to tell you that a well-shaken martini is a vast improvement over one that has settled and separated. A good mixture depends on understanding exactly how much to agitate a drink, as well as how quickly the ingredients will settle and if there are other mediating factors, such as temperature. If Bond really wanted to understand the science of his spirits, he could follow the examples of researchers who sent fluid mixture experiments to the International Space Station.
The Selectable Optical Diagnostics Instrument-Influence of Vibrations on Diffusion of Liquids, or SODI-IVIDIL, investigation addressed the question of fluid physics fundamentals while looking at how heat and particles move through liquids in microgravity. The scientists who conducted the space station investigation from October 2009 to January 2010 were not interested in cocktails, however, but instead wanted to verify current math models to predict liquid mixture behavior.
Researchers knew that studying reduced convection buoyancy – the transfer of heat by movement – aboard the space station could reveal fluid mixture behaviors hidden by gravity in experiments on the ground. Still, they needed to check if there were any side effects from the minor on-orbit tremors, known as g-jitter, such as crew movements or mechanical vibrations that could distort data.
To perform this series of experiments, researchers used the SODI optical instrument, which also helps with other station studies, such as SODI-Colloid. The European Space Agency, or ESA, built the SODI instrument for use aboard the station for fluids research in the space environment. The crew put the IVIDIL sample cells into SODI for processing and observation. The liquid binary solution samples, which are essentially fluids made up of a two-part mixture – similar to if Bond had vodka and water instead of his trademark martini – were then tested for their response to various vibrations.
After 55 repetitions of the experiment, researchers found that only major space station vibrations caused impacts, such as orbital debris avoidance maneuvers or dockings and undockings of spacecraft. The more common minor movements that are part of daily life aboard station did not influence the samples.
So what does this study mean for those of us on Earth, since we are not likely worried about Bond’s quest for the perfect martini in an orbital lounge? These results actually have direct applications to petroleum research.
Data from these space studies may help the oil industry generate formulas to predict correct measurements for the liquid to gas ratio in potential wells. This information aids geophysics and mineralogy experts as they evaluate the capacity of reservoirs – collections of natural resources that lay hidden in the ground. Using these formulas could prevent costly mistakes during exploration, leading to more accurate and affordable speculation.
Many things about liquids that we take for granted on earth can be very different in space. This creates problems for space mission planners. For instance, since It is nearly impossible to lift enough fuel for prolonged missions in one launch, scientists and engineers intend to store fuel and life support liquids, such as oxygen, water and ammonia, in depots in low-Earth, moon, or Mars orbits to provide ‘local’ supplies for longer crewed space flights.
However, for long-term storage in space it is difficult to predict the location of the liquid in the tank when it is time to withdraw it. Orbiting spacecraft travel in a low-gravity environment, due to the forces from the orbital motion balanced with the gravitational attraction of the moon, planet or sun. The resulting nearly weightless state prevents liquids from pooling at the bottom of a storage tank, and makes gas bubble locations nearly impossible to predict.
Engineers previously assumed the worst case, which was that the bubbles would disperse randomly throughout the tank. Such bubbles in the transmission lines would interrupt and interfere with the liquid flow and could lead to equipment failure. To solve this problem, scientists have begun using predictive computer modeling to determine the behavior of the liquid in the container. In one experiment the spacecraft was slowly rotated about its axis every 90 minutes. The slow rotation was enough to move the propellant to the top end of the tank, away from the bottom where the fluid would normally be located in a 1-gravity environment.
“This information is critical when trying to understand where the liquid is going to be located in the tanks,” said Greg Zimmerli with NASA’s Glenn Research Center, Cleveland. “SE-FIT can help determine where the liquid is located, which is critical to propellant management subsystems such as storage, liquid acquisition, and fuel gauging.”
Another problem is the state of liquid water in the vacuum of space. If you throw water out in space, about where we are in the solar system, any water that evaporates off it isn’t going to come back again – it’s a hard vacuum. It’s going to boil away and, as it does, it will get colder. At some point it might freeze,
but eventually it will sublime and turn into a gas. If you did the same thing where it’s very, very cold, out near Neptune or something, then it would just freeze. If you did get a bit of evaporation, it will cool down and freeze and essentially stay there as a lump of ice. If you use different liquids, different things will happen. Things like ionic liquids, they boil away so slowly that you could just have a blob of them which sat around as a liquid permanently.
So, if it’s not going to evaporate then the major force affecting it is surface tension, the only force which is left. So essentially, you get a huge droplet of water which is kind of held together by surface tension that would bobble around and just sit there, a perfect sphere, floating in space until someone drinks it or it hits something.
Thanks and attribution:
https://www.nasa.gov/mission_pages/station/research/news/Predict_Liquid2.html
https://www.thenakedscientists.com/articles/questions/how-do-liquids-behave-space
https://www.physicscentral.com/explore/action/fluids.cfm
https://peterengels.eu/portfolio/james-bond-actor-sean-connery-with-martini/
March 2024
Interesting Fact: Manufacturing in Space
Space is a dangerous place for humans: Microgravity sets our fluids wandering and weakens muscles, radiation tears through DNA and the harsh vacuum outside is an ever-present threat.
But for materials that show incredible strength, transmit information with barely any loss, form enormous crystals or even grow into organs, the harshness of space can be the perfect construction zone.
And as the cost of spaceflight goes down, more of these materials may become cost-effective to make or study in space. And soon, more and more people might be carrying around objects built off the planet.
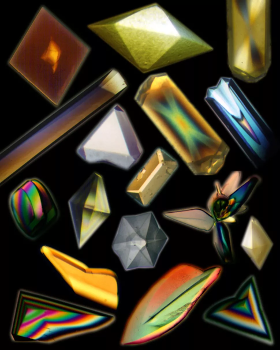
There are many aspects of space we can’t mimic on Earth,” says Dr. John Charles of NASA’s Johnson Space Center in Houston. “We can turn down air pressure in laboratory vacuum chambers and bombard samples with space-like radiation. But we can’t turn off gravity, or look down on Earth from above.”
Some experiments simply can’t be done on Earth. The space environment offers unique vacuum, temperature, pressure, radiation and gravity characteristics. Gravity of one-g is the natural environment for all terrestrial processes. And while higher g load levels can easily be produced and are common processing means, as in centrifugal separation, it is different with processing in a lower than one-g environment or weightlessness, because this condition can only be produced during free fall motions and is restricted to durations from fractions of seconds to about 30 seconds during the free fall trajectory flight in an airplane.
On earth only very few but remarkable manufacturing processes of very short processing cycle are able to use the lower than 1g level. An example is the free fall casting of lead shot, which was utilized centuries ago by pouring liquid lead through a screen atop the shot towers. Another example is the “atomizing” of metals and nonmetals to powders, tiny glass spheres and even hollow spheres called “microballoons”. However there is no process possible where a true equilibrium condition is reached until the free fall duration is extended to great length. This happens only in orbit where the satellite or complete manufacturing facility is consistently free falling around the earth.
The major importance of the sustained weightless environment lies in the fact that materials in liquid state become objects in their own right. From our terrestrial experience, liquids alone practically do not exist. They always need a container. The ever-present action of gravity causes buoyancy, separation and thermal convection during the interaction with other liquids, solids and gases, and is overshadowing and preventing many processes. Furthermore, on a macroscopic scale, molecular forces such as cohesion and adhesion do not play a large role, while in zero gravity they represent process controlling factors.
One example of the problems which arise during development in the weightless environment, was postulated to be the making of precise hollow spheres. It became obvious that the concentricity of the original gas pocket in the sphere is not self-adjusting because gas bubbles in a liquid in weightless environment stay where they are. It was found that application of acceleration will equalize the wall of the sphere. Acceleration of the wall mass generates a hydraulic pressure which causes the thicker portion to run into the thinner portion of the wall until the sphere has a uniform wall thickness. Radial acceleration can be caused by pulsing the environmental pressure which causes expansion and contraction of the sphere. Also, angular or translatory accelerations over two axis or planes will cause symmetric wall distribution. The spinning or translation can be induced during the electromagnetic container-less suspension of the material.
According to NASA’s In-Space Manufacturing (ISM) program, the ability to perform In-Space Manufacturing provides a solution towards sustainable, flexible missions (both in-transit and on-surface) through in-situ fabrication, repair, and recycling capabilities for critical systems, habitats, and mission logistics and maintenance. ISM is developing these capabilities by leveraging the highly disruptive technologies being developed terrestrially and adapting them for operations in the space environment.
According to Forbes magazine, innovators should not be limited to the tools they are given — or the planet they are on. On earth, we are limited to what we can engineer according to gravity, as well as the laws of nature and physics. But what can be manufactured in an environment that is not constricted in those ways?
Let your imagination take flight.
“We generally make things by subjecting them to a different environment,” said Andrew Rush, president and CEO of Made In Space, an in-space manufacturing company. “We make food by cooking it in fire, heating it up and causing chemical reactions. We make steel by heating things up at high temperature and maybe, depending on the steel, in a high-pressure environment. We can quench things; we can make things cold to make different materials or improve on those materials. Really, space-enabled materials are just another version of that, but instead of throwing something in a furnace and heating it to 1,000 degrees Fahrenheit [540 degrees Celsius] or something, we take it to space. In space, microgravity lets materials grow without encountering walls, and it allows them to mix evenly and hold together without traditional supports. And a nearby ultrahigh vacuum helps things form without impurities.”
Made in Space, Inc. has been delivering next-gen manufacturing capabilities in orbit to support exploration objectives and national security priorities. The company sent the first ceramic manufacturing facility to the International Space Station with the goal of demonstrating the ability to make turbine components with “higher strength and lower residual stress, due to a reduction in defects caused by gravity.” If successful, this effort will dramatically increase the commercial value of space manufacturing.
Manufacturing in microgravity and in manufacturing environments with unique gaseous compositions improves the manufacturing of metal alloys, for example, as elements can combine more consistently and efficiently to yield superior products with novel structures and properties.
Other manufacturing innovators envision a move to space as an excellent strategy for extending supply chain resiliency beyond current global boundaries. Bringing in a steady supply of electronic components and materials from earth to outer-space manufacturing plants is an opportunity to expand current footprints without the risk of climate-changing pollutants that are impacting earth. There’s also a great deal of interest in harnessing limitless solar power, as well as greater security and care using nuclear energy to further transform manufacturing in space.
And consider this:
When Galileo arrived at Jupiter in 1991, NASA scientists could not launch the spacecraft’s antenna completely, and several studies led them to conclude that cold welding had fused the antenna’s main mobile structure. What is cold welding?
If two pieces of the same type of metal touch in space they will permanently bond. This incredible fact is also known as “cold welding” and it happens because the atoms of two pieces of metal have no way of knowing they are separate. This doesn’t happen on Earth because of the air and water found between the pieces.
There are 3 kinds of bonds: covalent, ionic and metallic. In metallic bonds, metal atoms are kept close to each other so electrons can freely move between them. The attraction between these electrons and all of the metal nuclei keeps them held together. Metallic bonds are also responsible for the conductivity of metals. On earth, metals are almost always covered by a very thin film of oxide making metal-to-metal contact rare. In space there is no atmosphere to provide oxygen, which would renew the oxide coating. When the oxide wears off and metals are pressed together, there is true metal-to-metal contact. Since the metal surfaces are not microscopically smooth, the pressure is all taken out on a small number of microscopic high spots and the metals simply unite at these points.
You can try a similar effect in your own kitchen. Take a couple of ice cubes, let them warm up a bit, then press them together. They will weld into a single lump. Water already has all the oxygen in its molecule that it can accept, so it cannot oxidize further.
The effect has a lot of implications for spacecraft construction and the future of metal-based construction in vacuums.
Future human deep space exploration will be faced with the challenges of long mission endurance, as well as demand for robust surface infrastructure, increased solar power generation, and reliable high data rate communications, just to name a few. In-space manufacturing (ISM) can potentially address these challenges, and thus revolutionize human spaceflight.
Thanks and attribution:
https://www.nasa.gov/oem/inspacemanufacturing
https://www.sciencebyxanth.com/post/cold-welding-in-space
https://science.nasa.gov/science-news/science-at-nasa/2003/16jan_sts107/
Canaveral Society of Technical Societies, Space Congress Proceedings, Technology Today and Tomorrow,“Unique Manufacturing Processes in Space Environment”, 1970
https://www.space.com/40552-space-based-manufacturing-just-getting-started.html
January 2024
Interesting Fact: Don’t Just Stand There: Get Movin’
Every minute, you travel over 12,000 miles in space. That’s just while standing still. And thanks to the miracle of relativity, you’re not even aware of it. That’s because speed (velocity) is always measured relative to something else. If you are standing on earth looking up into the heavens, it appears to you that everything else is moving while you are standing still. But to someone on another planet or star, it would appear that you are moving and they are standing still.

Any speed you measure has to be relative to something that you are calling “stationary”.
For instance, a car speedometer tells you the speed of the car relative to the surface you are driving on. That would normally be the road, but maybe it is the deck of a ship that is steaming across the sea. Even standing still, the car is sitting on a planet that is rotating, and orbiting the Sun – the Sun is orbiting the galactic center and the whole galaxy is moving through the Virgo cluster of galaxies etc. So the car is only “stationary” relative to the surface of the earth.
Imagine that you are on a train, traveling at a steady speed of 50 miles per hour (mph). Your physics textbook on the table in front of you. Now, you and the textbook (and the train) are all moving at the same speed. To an outside observer standing next to the train tracks, you and the book are each rushing by at 50 mph. But, from your point of view, the book isn’t moving at all. That is, it’s not getting closer to or farther from you. The following are all true:
- You are moving at 50 mph relative to the observer next to the tracks.
- You are not moving, relative to the textbook.
- The book, the train, and yourself are not moving at all, relative to each other.
- Relative to the train, the ground is moving by at 50 mph.
- Relative to the ground, the train is moving by at 50 mph.
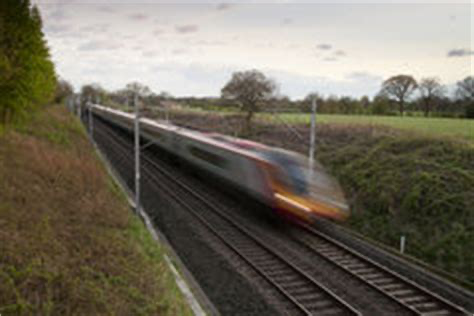
Bullet train in motion relative to the earth.
Sometimes people will try to impress others with how fast something is going. An example is jet airplanes flying in close formation. The announcer says they are only feet apart will moving at 400 miles per hour, or some such. But relative to others in the formation, they are traveling at close to zero mph. That is the important comparison, since they are fixated on maintaining exactly zero mph relative to the lead airplane.
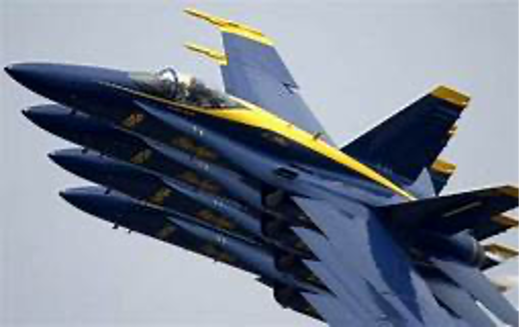
The Blue Angels in tight formation.
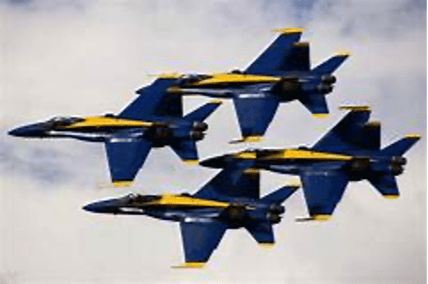
The Blue Angels in another tight formation.
The same can be said of astronauts in space. The ISS orbits the earth at a speed of 17,150 mph, yet the astronauts sense very little movement unless they look out the window. And even then, since that speed is relative to the earth, and the earth is so far away (approximately 220 statute miles), the apparent motion is minimal, akin to floating very slowly and gently.
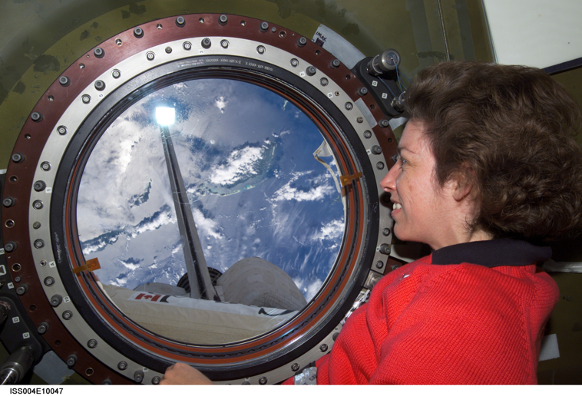
Astronaut Ellen Ochoa peers out window of International Space Station
(Courtesy NASA)
You can see the same effect with racing cars (or horses, or anything else). From the stands they are moving at 200 mph, while only a few inches from each other. But for the drivers, the relative motion is as close to zero as they can make it.
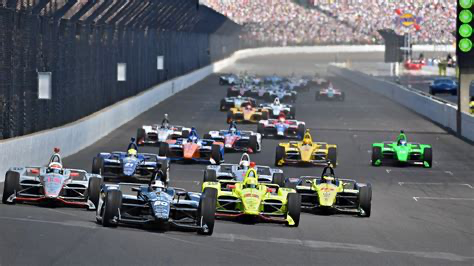
Indianapolis 500
Why is relative motion important?
Here’s an example from aviation.
During WWII, B-29’s over Japan encountered the jet stream, fierce winds above 25,000 feet that added or subtracted as much as 250 mph to an aircraft’s speed relative to the ground.
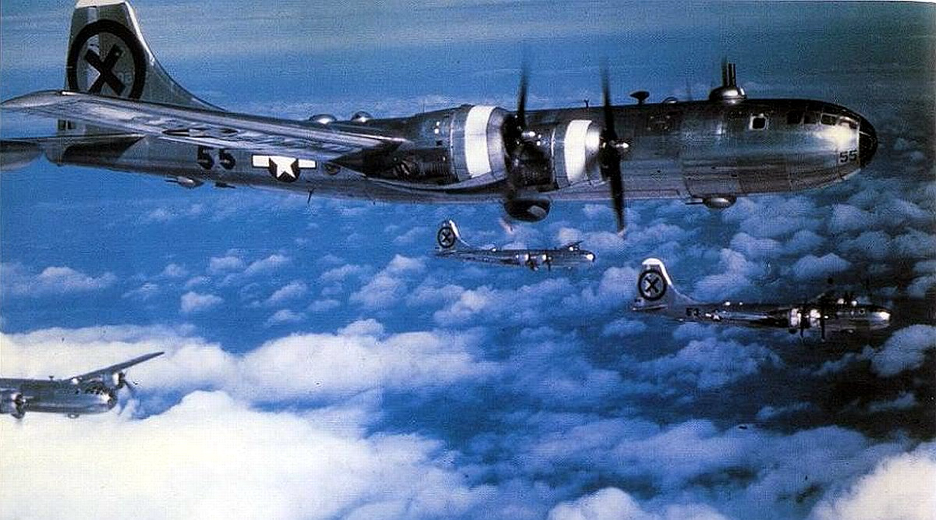
B-29 Superfortress
The B29 had the state-of-the-art bomb targeting system- the Norden Bombsight. The bomb system was an analog computer with 40+ parameters to tune, like wind velocity, the temperature outside, and even the earth’s curvature. The bombardiers (crew members who operated the device) would peek into their lens, mark the target, and set the parameters. The device would take care of the rest. The system worked flawlessly in Europe
But against Japan the jet stream pushed the bombers over the target too fast for the Norden bombsight to compensate. And flying against the jet stream on the return to base, the speed relative to the ground was so slow that the airplanes were sitting ducks or might even run out of fuel.
That is why all airplanes need two speedometers: one for airspeed (velocity through the air) and another for ground speed (velocity over the earth beneath the plane). In some cases, the jet stream exceeded the speed of the aircraft, causing it to actually track backwards with reference to the ground.
What about relative motion in space?
Earth is revolving on its axis, then around the sun, then around the center of the galaxy, etc. But how can we know how fast it is moving in space? We can’t. The laws of physics say it’s meaningless (impossible) to make a distinction between what is moving and what is not. However, if something is accelerating, unlike velocity, everybody in the Universe will agree that it is accelerating, but not by how much.
This is what happens when we say the Sun rises or the Sun sets. We say this because we tend to think earth is stationary and the sun is moving, just because our surroundings do not change but the sun appears to.
Einstein demonstrated this with trains. Suppose you are on a train that is stopped and another train is stopped beside it. If the other train begins moving forward, it is impossible for you to know whether that train is moving forward or if your train is moving backward.

Trains at station. Apparent motion is relative to the observer’s position.
Likewise, imagine you’re in a space suit, somewhere far from any other objects–so far away, you might as well be the only thing in the universe. Are you moving, and if so how fast? With nothing to compare your speed to, you can’t tell. Some people in history thought of space as a background against which you can be said to move or not move. But Galileo and Einstein, in their different ways, rejected this idea. They argued that since there’s no way to measure absolute motion (velocity relative to space itself), there’s no good way to define it, and so there’s no such thing.
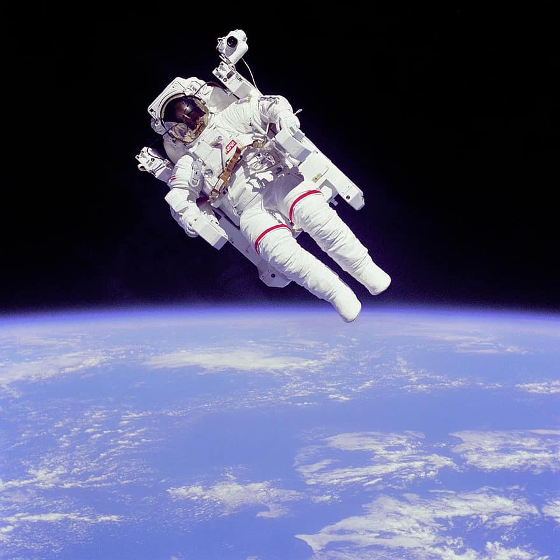
Astronaut Bruce McCandless on spacewalk. Is he moving?
We can only define velocity relative to another object. That object can be real or imaginary. It might just be a coordinate system we’ve invented. But it’s a free choice. There are infinitely many other coordinate systems we could have chosen to define our velocity relative to. If we chose one, such as the earth, and said “this is the still point relative to which everything else moves” it would just be an arbitrary human choice, a whim, and nothing directly to do with the real nature of motion in the universe. This is the viewpoint of Newtonian mechanics, based on Galileo’s ideas, and of Einstein’s relativity.
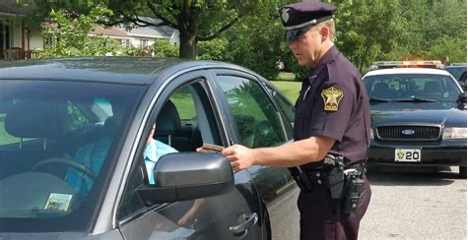
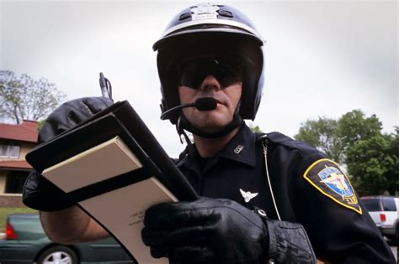

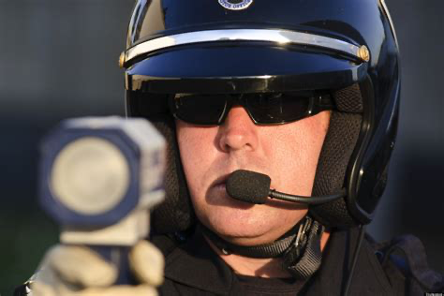
So the next time you get a speeding ticket, consider going to court and arguing that you weren’t actually moving at all. And because the law you were cited under probably did not identify a reference framework for measuring your speed, you are not guilty. Just hope you get a judge who has a degree in astrophysics. And a sense of humor! 🙂
Thanks and Attribution
https://www.quora.com/What-does-it-mean-by-speed-is-relative?share=1 (External Site: quora.com)
https://physics.stackexchange.com/questions/118858/what-does-relative-to-something-mean (External Site: physics.stackexchange.com)
https://byjus.com/jee/relative-motion/ (External site: byjus.com)
https://www.airspacemag.com/as-next/as-next-may-unbelievablebuttrue-180968355/ (External site: airspacemag.com)
https://medium.com/lessons-from-history/a-major-meteorological-discovery-in-the-midst-of-world-war-ii-jet-stream-4008187ad9b3 (External site: medium.com)
https://www.airforcemag.com/article/1008daylight/ (External site: airforcemag.com)
https://www.physicsforums.com/threads/velocity-in-space.416241/ (External site: physicsforums.com)
https://www.nasa.gov/topics/earth/images/index.html
https://www.dreamstime.com/stock-photography-fast-moving-trains-image12383722 (External site: dreamstime.com)
https://www.nasa.gov/content/astronaut-ellen-ochoa-looks-through-stations-destiny-lab-window
https://ww2colorfarbe.blogspot.com/2015/10/boeing-b-29-superfortress-flying-toward.html (External site: ww2colorfarbe.blogspot.com)