November 2023
Interesting Fact of the Month: What in the World is Dark Matter?
Astronomers are always on the hunt for dark matter, a mysterious form of matter that can’t be detected by normal means—hence its name. All of the universal matter that can be detected by current methods comprises only about 5 percent of the total matter in the universe. Dark matter makes up the rest, along with something known as dark energy. When people look at the night sky, no matter how many stars they see (and galaxies, if they’re using a telescope), they’re only witnessing a tiny fraction of what’s actually out there.
For the first 150 million years after the Big Bang, there were no galaxies or stars or planets. The universe was featureless. As time passed, the first stars formed. Stars collected into galaxies. Galaxies began to cluster together. Those clusters are made up of the galaxies and all the material between the galaxies. Clumps of matter smashed into each other and the planets in our solar system began to form around the sun. Obviously something must be holding everything: our solar system, galaxies and clusters of galaxies, together. And gravity is that something, the “glue” that holds the cosmos together.
Soon after Isaac Newton presented his theory of universal gravity, some astronomers began to speculate about the existence of objects that might emit little or no light, but could still be known by their gravitational tug on bright objects like stars and planets. This idea was strengthened in the 1700’s when Pierre Laplace argued that some objects might be massive enough to trap any light they emit (a simplistic idea of a black hole), and by the 1800’s Urbain Le Verrier and John Couch Adams used gravitational anomalies in the motion of Uranus to predict the presence of Neptune.
By this point astronomers had demonstrated the presence of dark nebulae seen only by the light they absorb from bright objects behind them. In some clusters the space between galaxies is filled with gas so hot that scientists cannot see it using visible light telescopes. The gas only can be seen as X-rays or gamma rays. Scientists look at that gas and measure how much there is between galaxies in clusters. By doing this, they discovered that there must be five times more material in the clusters than we can detect. It was clear that there was more in the Universe than could be seen by visible light. This invisible matter that we can’t detect is called “dark matter.”
The scientific story underlying dark matter is interesting. In the early 1900’s astronomers began to look at the spectra of galaxies. From this they could determine the speeds of stars as a function of their distance from the galactic center, known as a galactic rotation curve. Seen in visible light most galaxies have a bright center, dimming as you move away from the center. This would imply most of the stars (and thus most of the mass) is located near the center of a galaxy. If that’s the case, one would expect stars far from the center to move much more slowly than stars near the center, just as in our solar system Earth orbits the Sun much more quickly than distant Pluto. But when Max Wolf and Vesto Slipher measured the rotation curve of the Andromeda galaxy, they found it was basically flat, meaning that stars moved at the same speed regardless of their distance from galactic center.
One solution to this mystery was that Andromeda is surrounded by a halo of dark matter so that its mass is not concentrated in the center. While other galaxies showed similar rotation curves, seeming to support the presence of dark matter, even Fritz Zwicky was skeptical. Gas and dust within a galaxy might exert some kind of drag on fast moving stars, he argued, thus flattening the rotation curves. But by the 1950’s radio astronomy had progressed to the point where it could detect monatomic hydrogen through the famous 21 centimeter line. Radio observations of both the Andromeda galaxy and our own Milky Way galaxy showed similarly flat rotation curves. Since hydrogen is by far the most abundant element in the Universe, the results proved that not only stars, but the gas of any dark nebulae were orbiting the galaxies at similar speeds. Either galaxies contained significant dark matter or our understanding of gravity was very wrong.
This was such a radical notion that some astronomers began to question the validity of Newtonian gravity. By the 1980’s several alternative gravitational models were advanced, the most famous of which was Modified Newtonian Dynamics (MoND), proposed by Mordehai Milgrom. While these models did work well for things like dwarf galaxies, they worked horribly with things like galactic clusters. So other speculations and hypotheses were proposed.
One such was that black holes were the answer to the “dark matter” conundrum. That is, it was believed that the unaccounted for matter might be in black holes, and although the idea turns out not to be true, black holes continue to fascinate astronomers.
In 1998, two independent groups of researchers announced they had measured cosmic expansion to a higher degree of precision, and found that it was not slowing down, as expected, but getting faster. This acceleration implied some unknown force was counteracting gravity to make the universe expand at a greater rate.
Hubble Space Telescope (HST) observations of very distant supernovae have confirmed that a long time ago the universe was expanding more slowly than it is today. So the expansion of the universe has not been slowing due to gravity, as everyone thought, it has been accelerating. No one expected this, no one knew how to explain it. But something was causing it.
Eventually theorists came up with three sorts of explanations: Maybe it was a result of a long-discarded version of Einstein’s theory of gravity, one that contained what was called a “cosmological constant.” Maybe there was some strange kind of energy-fluid that filled space.
Maybe there is something wrong with Einstein’s theory of gravity and a new theory could include some kind of field that creates this cosmic acceleration.
Theorists still don’t know what the correct explanation is, but they have given the solution a name. It is called dark energy.
Despite the name, dark energy isn’t like dark matter, except that they’re both invisible. Dark matter pulls galaxies together, while dark energy pushes them apart.
Astronomers measure the expansion of the universe using the explosions of white dwarfs, called type Ia supernovas, which led to the discovery of dark energy in 1998. They also use thousands of galaxies to map sound waves, called baryon acoustic oscillations (BAO), produced when the universe was young, which stretch as the universe expands. Measurements show dark energy contributes about 68% of the total energy content of the universe.
Despite these scientific advances, where dark matter and dark energy are concerned, more is unknown than is known. We know how much dark energy there is because we know how it affects the universe’s expansion. Other than that, it is a complete mystery. But it is an important mystery. It turns out that roughly 68% of the universe is dark energy. Dark matter makes up about 27%. The rest – everything on Earth, everything ever observed with all of our instruments, all normal matter – adds up to less than 5% of the universe. Come to think of it, maybe it shouldn’t be called “normal” matter at all, since it is such a small fraction of the universe.
Another explanation for dark energy is that it is a new kind of dynamical energy fluid or field, something that fills all of space but something whose effect on the expansion of the universe is the opposite of that of matter and normal energy. Some theorists have named this fluid or field “quintessence,” after the fifth element of the Greek philosophers. But, if quintessence is the answer, we still don’t know what it is like, what it interacts with, or why it exists.
A last possibility is that Einstein’s theory of gravity is not correct. That would not only affect the expansion of the universe, but it would also affect the way that normal matter in galaxies and clusters of galaxies behaved. This fact would provide a way to decide if the solution to the dark energy problem is a new gravity theory or not: we could observe how galaxies come together in clusters. But if it does turn out that a new theory of gravity is needed, what kind of theory would it be? How could it correctly describe the motion of the bodies in the Solar System, as Einstein’s theory is known to do, and still give us the different prediction for the universe that we need? There are candidate theories, but none are compelling.
So the mystery continues.
In the meantime, thank Thomas Edison that at least here, in this tiny backwater of both the known and unknown universes, you can flip a switch and turn on the light!
Thanks and Attribution:
https://www.thoughtco.com/weird-and-amazing-astronomy-facts-3073144
https://www.nasa.gov/audience/forstudents/9-12/features/what-is-dark-matter.html
https://science.nasa.gov/astrophysics/focus-areas/what-is-dark-energy
https://www.cfa.harvard.edu/research/topic/dark-energy-and-dark-matter
Dark Matter and Dark Energy – Everything we know: Paperback – 2014, by Prof. M. Mork
September 2023
Interesting Fact of the Month: Strategies for Long Term Human Space Exploration
Human space exploration began on April 12, 1961 with the launch of Yuri Gagarin on Russia’s Vostok 1 rocket.
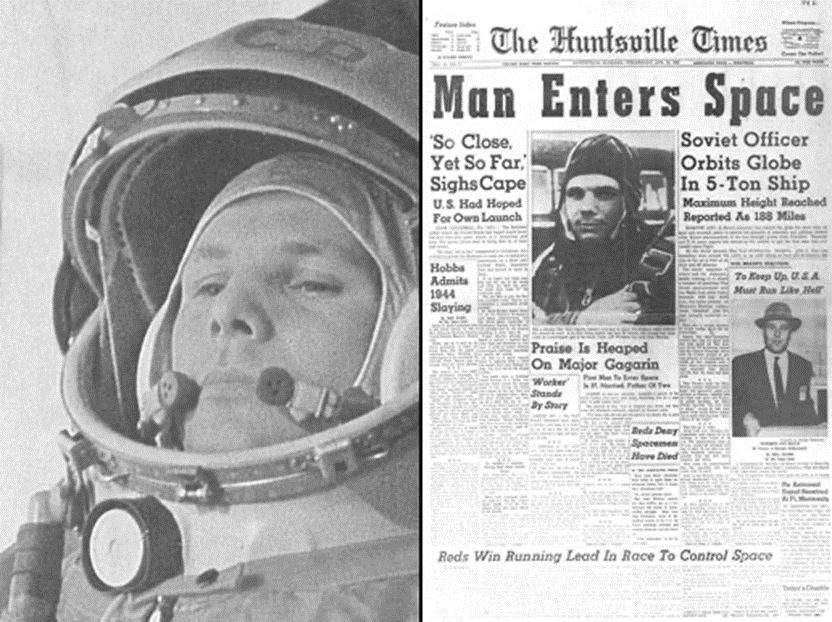
April 12 was already a huge day in space history twenty years before the launch of the first shuttle mission. On that day in 1961, Russian cosmonaut Yuri Gagarin (left, on the way to the launch pad) became the first human in space, making a 108-minute orbital flight in his Vostok 1 spacecraft. Newspapers like The Huntsville Times (right) trumpeted Gagarin’s accomplishment.
Since then, over 600 persons have ventured into space, some for only a few minutes, others for weeks or even months, and four of them for a year or more. Some have travelled to the moon and plans are underway for an eventual trip to Mars. But space is very large. A trip to Mars would take about seven months minimum, with another seven or more for the return. To get to Jupiter, with the idea to explore its moons, would take about five years each way. The European Space Agency plans to launch the Jupiter Icy Moons Mission in 2022, which will take nearly 8 years to reach Jupiter by 2030.
Our solar system features eight planets, seen in this artist’s diagram.
Our Solar System
A Solar System Family Portrait, from the Inside Out
How long does it take to get to Saturn? That question happens to have several answers. Just as it can take different amounts of time to get to a destination here on Earth depending on what route you take it can take different amounts of time to get to Saturn based on how you travel.
In the past spacecraft have taken greatly different amounts of time to make it to Saturn. Pioneer 11 took six and a half years to arrive. Voyager 1 took three years and two months, Voyager 2 took four years, and the Cassini spacecraft took six years and nine months to arrive. The New Horizons spacecraft took a short two years and four months to arrive on the scene.
Why such huge differences in flight time?
The first factor to consider is whether the spacecraft is launched directly toward Saturn or if the spacecraft is sent toward other celestial objects to use their gravity to slingshot itself to Saturn. Another factor is consider is the type of engine propelling the spacecraft, and a third factor to think about is that it takes a great deal of time to slow down, so if a spacecraft is simply going to flyby, it need not slowdown, but if it is to orbit, its trip to Saturn will take longer.
And these are just our closest neighbors in space. To get to the closest galaxy to ours, the Canis Major Dwarf, would take approximately 749,000,000 years to travel the distance of 25,000 light
years! That means if we could travel at the speed of light, it would still take 25,000 years! It would appear that sending human astronauts to the far reaches of our own solar system, let alone beyond, would take inordinate amounts of time. So, what are some strategies for overcoming this seemingly impossible problem?
Space scientist Ian Crawford, professor of planetary science and astrobiology at Birkbeck College in the United Kingdom concedes that even with super-fast rockets traveling at speeds of 10 percent or more of the speed of light, it would take many human lifetimes to reach all but the very nearest stars. And although he is an advocate of human exploration of the solar system, Crawford says that interstellar distances are too vast to make a human voyage conceivable within the next few hundred years.
“I think humans can explore the planets more effectively than robots, and I also think there are cultural reasons for sending humans into space, to broaden our range of experiences and enrich human culture,” he said. “Now, it’s true that all of that would apply on the interstellar scale as well — it’s just that the distances are so large and the technical difficulties so great that advocating it at this stage is almost inconceivable.”
Even so, several ideas exist for what are known as “Slow Boat” concepts that might one day take humans to the stars, including:
Sleeper Ships, in which human crewmembers are kept in a state of “deep sleep” or “suspended animation” for the duration of the very long voyage. This idea has featured in several science fiction movies, including Stanley Kubrick’s “2001: A Space Odyssey” made in 1969, Ridley Scott’s “Alien” in 1979, and James Cameron’s “Avatar” in 2009.
World Ships, also known as generation ships or interstellar arks, would be giant self-contained space habitats carrying large populations of humans and other species from Earth on a relatively leisurely journey to colonize exoplanets — journeys that would take many centuries to complete. Entire generations would live and die during the voyage, and only the descendants of the original population would arrive at the destination.
Embryo Ships would send cryogenically frozen embryonic humans, instead of sleeping or living humans, to a distant colony planet, where they would be “hatched out” and educated on their mission by a crew of protective robots.
Faster than light?
Wherever astronomers look in the universe, the Theory of Relativity holds fast. As Albert Einstein showed, it’s not possible to accelerate a mass to the speed of light in space, nor past it.
But Einstein’s equations may yet hold a few tricks that could someday let science do an end-run around the known laws of physics and achieve faster-than-light (FTL) travel — a Holy Grail for generations of science fiction fans.
The best-known scientific concept for FTL travel is the Alcubierre drive, proposed by theoretical physicist Miguel Alcubierre, Director of the Nuclear Sciences Institute at the National Autonomous University of Mexico (UNAM) in 1994. The proposed drive operates by using intense gravitational forces, generated by two rotating rings of dense exotic matter, to shrink the physical dimensions of space in front of the spacecraft while expanding the space behind it, at a rate that could appear to exceed the speed of light.
In Alcubierre’s proposal, which requires a type of exotic matter for the rings that is not known to exist, the spacecraft inside the “warp bubble” created by the drive would never travel faster than light in its local space, and so would not violate the laws of relativity.
Other speculative ideas for faster-than-light (FTL) travel include using trans-dimensional wormholes — also theoretically possible, but not known to exist — to travel between entangled but distant regions of space; or to daringly skim the edges of a large, spinning black hole, as depicted in Christopher Nolan’s 2014 movie “Interstellar.”
But Crawford notes that the concept of faster-than-light travel is rife with unknowns and apparent contradictions, such as violating the principle of causality, in which events are caused by other events that happened earlier in time, and not the other way around. So, it’s likely the proposals would prove impossible, even if they were technologically feasible to attempt. “I don’t want to sound overly pessimistic, because I can see huge benefits of being able to travel to the stars, but the laws of physics are the laws of physics, and it is going to be really difficult,” Crawford said.
Thanks and Attribution:
https://www.nasa.gov/mission_pages/shuttle/sts1/gagarin_anniversary.html
https://solarsystem.nasa.gov/solar-system/our-solar-system/galleries
https://mars.nasa.gov/mars2020/timeline/cruise/
https://www.universetoday.com/128259/long-take-get-jupiter/
https://www.universetoday.com/15312/how-long-does-it-take-to-get-to-saturn/
https://imagine.gsfc.nasa.gov/features/cosmic/nearest_galaxy_info.html
https://www.livescience.com/55981-futuristic-spacecraft-for-interstellar-space-travel.html
https://duckduckgo.com/?q=picture+of+Canis+Major+Dwarf+Galaxy&t=chromentp&atb=v225-1&ia=web
https://www.space.com/how-detect-wormholes-supermassive-black-hole.html
June 2023
Interesting Fact: How many moons are there?
We all know that earth has only one moon, right? After all, we can see it, and track it across the sky, even predict its appearances. So there is only one. Sometimes it appears as a quarter moon, sometimes a half moon, sometimes a full moon, and sometimes a new moon. But it’s all the same moon.
Yes, but from time immemorial earthlings have given many different names to their moon. For example there is a Blue Moon. There are two different definitions for a Blue Moon. A seasonal Blue Moon is the third Full Moon of an astronomical season that has four Full Moons. A monthly Blue Moon is the second Full Moon in a calendar month with two Full Moons.
The historical origins of the term and its two definitions are shrouded in a bit of mystery and, by many accounts, an interpretation error. Some believe that the term “blue moon” meaning something rare may have originated from when smoke and ashes after a volcanic eruption turned the Moon blue.
Some Moon names came from Native American culture and differed from tribe to tribe. The full moon names were passed down through generations and adopted by Colonial Americans.
For example, each month received a name for its full appearance:
January – Full Wolf Moon. Wolves were often heard howling during this time of year, due to gathering for hunting, locating pack members, and defining territory. Alternative names include Canada Goose Moon, Center Moon, Cold Moon, Great Moon, Spirit Moon.
Full Wolf Moon
February – Full Snow Moon. February is a month when it snows quite a lot, so that’s where that name came from! Alternative names include Bald Eagle Moon, Bear Moon, Eagle Moon, Groundhog Moon.
Full Snow Moon
March – Full Worm Moon. This moon name refers to the “worm larvae” that came from tree bark that emerged after hiding in winter. Alternative names include Eagle Moon, Goose Moon, Snow Crust Moon, and Wind Strong Moon.
The full moon rises behind the Church of our Lady in Munich, Germany, Sunday, March 28, 2021. The full moon in March is called the “Worm Moon.” (AP Photo/Matthias Schrader)
April – Full Pink Moon. This name came from the “moss pink” spring wildflower that starts to bloom. Alternative names include Breaking Ice Moon, Frog Moon, Moon of the Red Grass Appearing, Moon When The Streams Are Again, Sugar Maker Moon.
Full Pink Moon
May – Full Flower Moon. Spring is here and all the flowers are blooming! Alternative names include Budding Moon, Egg Moon, Milk Moon, Leaf Budding Moon, Frog Moon, Planting Moon.
Super Flower Moon
June – Full Strawberry Moon. This is the time when gathering ripening strawberries is the best (especially in the northeastern United States). Alternative names include Berries Ripen Moon, Birth Moon, Blooming Moon, Hot Moon, Green Corn Moon, Flower Moon.
Strawberry Moon (Summer Solstice Full Moon) 2021
Credits: Space.com
July – Full Buck Moon. At this time of year, a male deer’s antlers (a buck) are in full growth. Alternative names include Berry Moon, Halfway Summer Moon, Hay Moon, Moon of the Ripe Corn Moon, Thunder Moon.
Full Buck Moon
August – Full Sturgeon Moon. Sturgeon is a fish that is caught frequently during this time in the Great Lakes. Alternative names include Black Cherries Moon, Corn Moon, Grain Moon, Flying Up Moon.
Full Sturgeon Moon
Credits: Mentalfloss.com
September – Full Corn Moon. This name refers to the time when it’s best to harvest the corn, which is why it is also considered to be called Harvest Moon. Alternative names include Autumn Moon, Moon of Brown Leaves, Mating Moon, and Leaves Turning Moon.
Full Corn Moon
September 2020 Full Corn Moon brightens the night sky The full moon rises behind the Byzantine-era Hagia Sophia in the historic Sultanahmet district of Istanbul, early Tuesday, Sept. 1, 2020. (AP Photo/Emrah Gurel)
October – Full Hunter’s Moon. This is the month of hunting and laying in provisions for the winter months that are approaching. Alternative names include Drying Rice Moon, Falling Leaves Moon, Migrating Moon.
Full Hunter’s Moon
November – Full Beaver Moon. This name refers to when beavers finished preparing for winter and start retreating into their homes. Alternative names include Deer Rutting Mon, Freezing Moon, Whitefish Moon.
NASA welcomes spectacular Beaver Moon, 2019
Credits: express.co.uk
December – Full Cold Moon. This name refers to when the nights become long, dark, and very, very cold. Alternative names include Frost Exploding Trees Moon, Long Night Moon, Mid-winter Moon, Snow Moon.
December’s Full Cold Moon 2019
Credits: returntonow.mnet
These moon names primarily refer to the months and seasons in the northern hemisphere. In the southern hemisphere, however, the seasons are reversed, and just reversing the names doesn’t work because some of the referenced animals or phenomena don’t exist in that half of the world. Also, their names aren’t consistent between countries, regions, cultures, even tribes. Herewith a list from <lunarphasepro.com>:
Chinese
January | Holiday Moon |
July | Hungry Ghost Moon |
February | Budding Moon |
August | Harvest Moon |
March | Sleepy Moon |
September | Chrysanthemum Moon |
April | Peony Moon |
October | Kindly Moon |
May | Dragon Moon |
November | White Moon |
June | Lotus Moon |
December | Bitter Moon |
Celtic
January | Quiet Moon |
July | Moon of Claiming |
February | Moon of Ice |
August | Dispute Moon |
March | Moon of Winds |
September | Singing Moon |
April | Growing Moon |
October | Harvest Moon |
May | Bright Moon |
November | Dark Moon |
June | Moon of Horses |
December | Cold Moon |
English Medieval
January | Wolf Moon |
July | Mead Moon |
February | Storm Moon |
August | Corn Moon |
March | Chaste Moon |
September | Barley Moon |
April | Seed Moon |
October | Blood Moon |
May | Hare Moon |
November | Snow Moon |
June | Dyan Moon |
December | Oak Moon |
Neo Pagan
January | Ice Moon |
July | Rose Moon |
February | Snow Moon |
August | Lightening Moon |
March | Death Moon |
September | Harvest Moon |
April | Awakening Moon |
October | Blood Moon |
May | Grass Moon |
November | Tree Moon |
June | Planting Moon |
December | Long Night Moon |
New Guinea
Name | Rainbow Fish Moon |
Name | Black Trevally Moon |
Name | Parriotfish Moon |
Name | Open Sea Moon |
Name | Palolo Worm Moon |
Name | Tiger Shark Moon |
Name | Flying Fish Moon |
Name | Rain & Wind Moon |
American Indian (Cherokee)
January | Ice Moon |
July | Ripe Corn Moon |
February | Bony Moon |
August | Fruit Moon |
March | Windy Moon |
September | Nut Moon |
April | Flower Moon |
October | Harvest Moon |
May | Planting Moon |
November | Trading Moon |
June | Green Corn Moon |
December | Snow Moon |
American Indian (Choctaw)
January | Cooking Moon |
July | Crane Moon |
February | Little Famine Moon |
August | Women’s Moon |
March | Big Famine Moon |
September | Mulberry Moon |
April | Wildcat Moon |
October | Blackberry Moon |
May | Panther Moon |
November | Sassafras Moon |
June | Windy Moon |
December | Peach Moon |
American Indian (Other)
January | Wolf Moon |
July | Buck Moon |
February | Snow Moon |
August | Sturgeon Moon |
March | Worm Moon |
September | Corn/Harvest Moon |
April | Pink Moon |
October | Hunter/Harvest Moon |
May | Flower Moon |
November | Beaver Moon |
June | Strawberry Moon |
December | Cold/Long Nights Moon |
American Indian (Dakotah Sioux)
January | Moon of the Terrible |
February | Moon of the Raccoon, Moon When Trees Pop |
March | Moon When Eyes Are Sore from Bright Snow |
April | Moon When Geese Return in Scattered Formation |
May | Moon When Leaves Are Green, Moon To Plant |
June | Moon When June Berries Are Ripe |
July | Moon of the Middle Summer |
August | Moon When All Things Ripen |
September | Moon When The Calves Grow Hair |
October | Moon When Quilling and Beading is Done |
November | Moon When Horns Are Broken Off |
December | Twelfth Moon |
Colonial American
January | Winter Moon |
July | Summer Moon |
February | Trapper’s Moon |
August | Dog Day’s Moon |
March | Fish Moon |
September | Harvest Moon |
April | Planter’s Moon |
October | Hunter’s Moon |
May | Milk Moon |
November | Beaver Moon |
June | Rose Moon |
December | Christmas Moon |
North American
January | Old Moon |
July | Thunder Moon |
February | Snow Moon |
August | Green Moon |
March | Sap Moon |
September | Fruit Moon |
April | Grass Moon |
October | Harvest Moon |
May | Flower Moon |
November | Hunter’s Moon |
June | Rose Moon |
December | Cold Moon |
Wiccan
January | Wolf Moon |
July | Mead Moon |
February | Storm Moon |
August | Wyrt Moon |
March | Chaste Moon |
September | Barley Moon |
April | Seed Moon |
October | Blood Moon |
May | Hare Moon |
November | Snow Moon |
June | Dyad Moon |
December | Oak Moon |
Southern Hemisphere
January | Hay Moon, Buck Moon, Thunder Moon, Mead Moon |
February | Grain Moon, Sturgeon Moon, Red Moon, Wyrt Moon, Corn Moon, Dog Moon, Barley Moon |
March | Harvest Moon, Corn Moon |
April | Harvest Moon, Hunter’s Moon, Blood Moon |
May | Hunter’s Moon, Beaver Moon, Frost Moon |
June | Oak Moon, Cold Moon, Long Night’s Moon |
July | Wolf Moon, Old Moon, Ice Moon |
August | Snow Moon, Storm Moon, Hunger Moon, Wolf Moon |
September | Worm Moon, Lenten Moon, Crow Moon, Sugar Moon, Chaste Moon, Sap Moon |
October | Egg Moon, Fish Moon, Seed Moon, Pink Moon, Waking Moon |
November | Corn Moon, Milk Moon, Flower Moon, Hare Moon |
December | Strawberry Moon, Honey Moon, Rose Moon |
South African
January | Thunder Moon |
July | Old Moon |
February | Grain Moon |
August | Hunger Moon |
March | Harvest Moon |
September | Storm Moon |
April | Hunter’s Moon |
October | Seed Moon |
May | Fell Moon |
November | Flower Moon |
June | Cold Moon |
December | Fruit Moon |
The Nordic as well as the Mayan civilizations had their own names for moons, which may not yet have been translated out of their own languages.
Viking Moon
Credits: deviantart.com
So how many moons are there? Apparently as many as peoples, tribes, and civilizations are willing to apply names to. Surely more than a hundred. Maybe even a thousand!
Thanks and Attribution:
https://www.thefactsite.com/wolf-moon-facts/
https://thoughtcatalog.com/january-nelson/2021/02/moon-names/
https://www.timeanddate.com/astronomy/moon/blue-moon.html
https://thoughtcatalog.com/january-nelson/2021/02/moon-names/
https://www.lunarphasepro.com/full-moon-names/
https://weather.com/photos/news/2021-03-29-super-worm-moon-photos
https://www.moonomens.com/super-flower-moon/
https://orangectlive.com/2017/07/08/full-buck-moon-at-peak-just-after-midnight/
https://www.mentalfloss.com/article/596320/how-to-see-the-full-sturgeon-moon-august
https://www.whats-your-sign.com/native-american-moon-signs.html
https://www.express.co.uk/news/science/1203049/November-Full-Moon-NASA-welcomes-Beaver-Moon-2019
https://returntonow.net/2019/12/05/decembers-full-cold-moon-peaks-at-1212-a-m-on-12-12/
May 2023
Interesting Fact of the Month: An Astronaut’s Best Friend(s)
Space is a hostile environment and before astronauts were allowed to venture there, animals were employed to determine whether the environment could be made safe for human beings. The best known was a dog, a three year old stray mutt with the name Laika (Russian for “barker”) who became the first living creature to enter orbit on November 3, 1957 aboard Sputnik 2. She may have been man’s best friend, but the Russian’s weren’t hers, having omitted a re-entry plan and allowing her to die in space as well. Although Laika died only hours after launch, data returned from her flight proved that it was possible for a living being to enter space.
Laika, the Russian space dog
Credits: Bettmann/Contributor/Bettmann/Getty Image
On 22 July 1951, the Soviet Union launched the R-1 IIIA-1 flight, carrying the dogs Tsygan (Russian for “Gypsy”) and Dezik into space, but not into orbit. These two dogs were the first living higher organisms successfully recovered from a spaceflight. Both space dogs survived the flight, although Dezik would die on a subsequent flight. But dogs weren’t the only animals pressed into service to pave the way for safe human space flight.
On February 20, 1947 fruit flies were launched by the U.S. aboard a V-2 rocket. They were more fortunate than Laika: their capsule parachuted back to earth and they were recovered alive. The purpose of their mission was to explore the effects of cosmic radiation exposure at high altitudes. Fruit flies were chosen because they were genetically similar to humans.
The first monkey, first primate, and first mammal in space was Albert II, a rhesus monkey, also launched on a V-2 rocket, on June 14, 1949. Unfortunately his parachute failed and he died on impact after a mission that lifted him to an altitude of 83 miles, but not into orbit. Dozens of other monkeys and apes were implanted with sensors to measure vital signs, and launched into space to study the forces and conditions astronauts would be subject to in the future.
On 31 January 1961, Ham, a chimpanzee, was launched in a Mercury capsule aboard a Redstone rocket to become the first great ape in space. Ham’s mission was Mercury-Redstone 2. The chimpanzee had been trained to pull levers to receive rewards of banana pellets and avoid electric shocks. His flight demonstrated the ability to perform tasks during spaceflight.
A little over three months later the United States sent Alan Shepard into space.
Enos became the first and only chimpanzee in orbit on 29 November 1961, in another Mercury capsule, an Atlas rocket, Mercury-Atlas 5. Enos completed more than 1,250 training hours at the University of Kentucky and Holloman Air Force Base. Training was more intense for him than for his predecessor Ham, who had become the first great ape in space in January, 1961, because Enos was exposed to weightlessness and higher G’s for longer periods of time. His training included psychomotor instruction and aircraft flights.
Enos was selected for his Project Mercury flight only three days before launch. Two months prior, NASA launched Mercury-Atlas 4 on September 13, 1961, to conduct an identical mission with a “crewman simulator” on board. Enos flew into space aboard Mercury-Atlas 5, completing his first orbit in 1 hour and 28.5 minutes.
Enos was scheduled to complete three orbits, but the mission was aborted after two due to two issues: capsule overheating and a malfunctioning “avoidance conditioning” test subjecting the primate to 76 electrical shocks. The capsule was brought aboard USS Stormes in the late afternoon and Enos was immediately taken below deck by his Air Force handlers. Enos’s flight was a full dress rehearsal for the next Mercury launch on February 20, 1962, which would make John Glenn the first American to orbit Earth, after astronauts Alan Shepard, Jr. and Gus Grissom’s successful suborbital space flights.
On 31 August 1950, the U.S. launched a mouse into space aboard a V-2, but the rocket disintegrated because the parachute system failed. The U.S. subsequently launched many mice to study how quickly they adapt to microgravity conditions. The R-1, a Soviet copy of the German V-2 also carried mice into space, but insulated electrical wiring attracted the vermin. In one January 1953 incident, thousands of flood-displaced mice disabled many rockets by eating the insulation, requiring “hundreds of cats and repairmen”
“Mouse in Space”
Credits: NYPost.com
On 13 December 1958, a squirrel monkey named Gordo was launched from Cape Canaveral, Florida, Although the nose cone recovery parachute failed to operate and Gordo was lost, telemetry data sent back during the flight showed that the monkey survived the 10 g of launch, 8 minutes of weightlessness and 40 g of reentry at 9000 mph). The nose cone sank 1,302 nautical miles downrange from Cape Canaveral and was not recovered. He died on splashdown when a flotation mechanism failed, but Navy doctors said signals on his respiration and heartbeat proved humans could withstand a similar trip.
Able and Baker became the first monkeys to survive spaceflight after their 1959 flight. On 28 May 1959, aboard Jupiter IRBM AM-18, were a 7 lb American-born rhesus monkey, Able, and an 11 oz squirrel monkey from Peru, Baker. The monkeys rode in the nose cone of the missile to an altitude of 360 miles and a distance of 1,699 miles down the Atlantic Missile Range from Cape Canaveral, Florida. They withstood forces 38 times the normal pull of gravity and were weightless for about 9 minutes. A top speed of 9,900 mph was reached during their 16-minute flight. The monkeys survived the flight in good condition but Able died four days after the flight from a reaction to anesthesia, while undergoing surgery to remove an infected medical electrode. Baker was the center of media attention for the next several months as she was watched closely for any ill-effects from her space flight. She was even mated in an attempt to test her reproductive system. Baker lived until 29 November 1984, at the US Space and Rocket Center in Huntsville, Alabama.
Monkeys Abel and Baker on Life Magazine cover
On 2 July 1959, a launch of a Soviet R2 rocket, which reached 132 miles, carried Marfusha, the first rabbit to go into space. One of the problems of using rabbits is their high susceptibility to stress.
A 19 September 1959 launch of Jupiter AM-23, carried 2 frogs along with 12 mice but the rocket was destroyed during launch. The frogs were part of an attempt to study the effects of weightlessness upon the inner-ear balance mechanism.
Amphibians, especially frogs, have been helping make one great leap for mankind since 1959. However, the most significant frog flight came in 1970 when NASA launched the Orbiting Frog Olotith spacecraft, containing two bullfrogs. The word ‘olotith’ refers to the frogs’ inner-ear balance mechanism and the experiment was designed to investigate the effect of space travel on motion sickness. Electrodes were implanted into the frogs’ thoraxes and the vestibular system within the ear to record data on the effects of sustained weightlessness. The study found that after 6 days the frogs acclimatized and their vestibular system had returned to normal.
The Space Shuttle’s Oddest Passengers
Credits: wired.com
In 1968, the race was on between the USA and the Soviet Union to get a man on the moon. The Russians launched the Zond 5 spaceship with a capsule carrying samples of soil and seeds, some worms and two steppe tortoises. The tortoises completed a circuit around the moon and after six days returned to Earth. Though the plan had been for Zond 5 to land in Kazakhstan, the capsule veered off course and was eventually retrieved from the Indian Ocean. Thankfully, the tortoises were still alive, though they had lost weight by 10 per cent.
In 1973 NASA was keen to observe the effects of microgravity on animals that moved three dimensionally on Earth. The human astronauts suffered from space sickness, and likewise the fish swam in loops rather than straight lines. In a few days, both the astronauts and aquanauts had got their bearings.
Zebrafish flex their muscles for research aboard the ISS
Credits: Phys.org
Also In 1973, two garden spiders called Anita and Arabella, were used in an experiment to see if they could still spin webs in space. The experiment was the brain-child of Massachusetts high school student Judith Miles. Both spiders managed to spin webs, even though the webs were slightly finer than on Earth. The study revealed a great deal about the effects of microgravity on motor response.
Spiders in Space – The Experiment Photograph by James Temple
Credits: Fineartamerica.com
In 2012, the Japanese space agency decided to send fish up to the International Space Station. Their aquarium had an automatic feeding system, a water circulation system, and LED lights to represent day and night. The fish chosen to go up were medaka, which have transparent skin, making it easier for researchers to see what was going on inside the fish. The purpose of the experiment was to see how fish would respond to radiation impact, bone degradation and muscle wastage.
Laika Monument, Moscow, Russia
These and numerous other animal experiments in space travel were primarily carried out to enhance the safety of the human astronauts and crews who would follow. Not only the animals mentioned but others, including cats, rats, bullfrogs, tortoises, parasitic wasps, flour beetles, newts, meal worms, frog eggs, fish, bats, cockroaches, spiders, scorpions, crickets, snails, bacteria, amoeba, plants, and fungi, were carried aloft by scientists from the U.S., Russia, France, Argentina, China, and Japan. Regardless of how we view their involuntary sacrifices, we should be grateful for their contribution to the explorations that have followed in the 60+ years since these missions were performed.
Unfortunately, few of them have been formally honored, although Laika is remembered with a monument in Moscow and Ham with a grave at the Museum of Space History in Alamogordo, New Mexico.
Thanks and attribution:
https://www.thoughtco.com/laika-the-dog-1779334
Rosenberg, Jennifer. “Laika, the First Animal in Outer Space.” ThoughtCo, Sep. 1, 2021, thoughtco.com/laika-the-dog-1779334.
https://en.wikipedia.org/wiki/R-1_(missile)
https://en.wikipedia.org/wiki/Soviet_space_dogs
https://www.toptenz.net/top-10-animals-astronauts-in-space.php
https://en.wikipedia.org/wiki/Enos_(chimpanzee)
https://www.discoverwildlife.com/animal-facts/animals-in-space/
https://history.nasa.gov/animals.html
https://www.atlasobscura.com/places/laika-monument
https://flashbak.com/wp-content/uploads/2012/10/ham-astrochimp.jpeg
https://i.ytimg.com/vi/w2HrglMnLZI/hqdefault.jpg
https://phys.org/news/2015-02-zebrafish-flex-muscles-aboard-international.html
April 2023
Cecilia Payne: The most important space scientist you never heard of
Today, we know that the universe is mostly made of hydrogen and helium. However, the person who discovered what stars are made of never got the accolades she deserved. In honor of Women’s History Month, here is the story of Cecilia Payne, one of the most important space scientists you never heard of.
Cecilia Payne was a renowned astrophysicist who is best known for her groundbreaking work on the composition of the sun. Her work has had a profound impact on our understanding of the universe, and her discoveries continue to inspire new generations of scientists. However, Payne’s path to scientific discovery was not an easy one. She faced numerous challenges and obstacles due to her gender, but she persevered and became a trailblazer in her field.
Cecilia Payne was born on May 10, 1900, in Wendover, England. From a young age, she showed an aptitude for science, particularly chemistry. Her parents were supportive of her interests, and they encouraged her to pursue her studies. Payne attended St Paul’s Girls’ School in London, where she excelled in her studies and developed a passion for astronomy.
Newnham College, Cambridge, circa 1920
In 1919, Payne was awarded a scholarship to study at Newnham College, Cambridge. She was one of the first women to study astronomy at the university, and she quickly impressed her professors with her intelligence and work ethic. However, she faced significant challenges as a woman in a male-dominated field. At the time, women were not allowed to receive degrees from Cambridge, so Payne was awarded a certificate instead. Despite this setback, she continued to pursue her studies and eventually earned a Ph.D. in astronomy from Radcliffe College in the United States.
In her Ph.D. thesis (published as Stellar Atmospheres [1925]), Payne used the spectral lines of many different elements and the work of Indian astrophysicist Meghnad Saha, who had discovered an equation relating the ionization states of an element in a star to the temperature to definitively establish that the spectral sequence did correspond to quantifiable stellar temperatures. Payne also determined that stars are composed mostly of hydrogen and helium, a breakthrough discovery that would change our understanding of the universe. Astronomers Otto Struve and Velta Zebergs later called her thesis “undoubtedly the most brilliant Ph.D. thesis ever written in astronomy.”
Payne’s discovery was initially met with skepticism and disbelief, as many scientists found it hard to believe that the most abundant element in the universe was one that had been overlooked for so long. This was a significant departure from the prevailing theory, which held that stars were made up of the same elements as the Earth. However, her findings were eventually accepted and have since become a cornerstone of astrophysics.
After completing her studies, Payne began her career as an astronomer. She worked at Harvard University, where she was one of only a handful of women in the department, again facing discrimination from her male colleagues, who often dismissed her work and ideas. However, she persevered and continued to pursue her research.
Despite her groundbreaking work Payne was often paid less than her male colleagues, and she was denied the title of professor, despite being one of the most prominent scientists in her department. She was also excluded from many academic and social events, as women were not allowed to participate in them at the time.
However, she remained committed to her work and continued to make groundbreaking discoveries, receiving numerous honors and awards throughout her career, including the Henry Norris Russell Prize, considered one of the most prestigious awards in astrophysics. She also became the first woman to be appointed a full professor at Harvard University in 1956, paving the way for future generations of women in science.
Cecilia Payne’s legacy continues to inspire and influence new generations of scientists. Her work on the composition of the sun has had a profound impact on our understanding of the universe, and her perseverance in the face of discrimination and sexism serves as an inspiration to many. Despite the challenges she faced, Payne never lost her passion for science and remained committed to her work throughout her life.
Cecilia Payne was indeed a trailblazing scientist who made significant contributions to our understanding of the universe. Her work on the composition of the sun was groundbreaking and has had a lasting impact on the field of astrophysics. Despite the challenges she achieved notable accomplishments as a pioneering woman in the field, among them:
Payne was the first person to receive a Ph.D. in astronomy from Radcliffe College. She wrote her dissertation on the spectra of stars, which laid the groundwork for her later discoveries.
Payne’s initial discovery on the composition of stars was so radical at the time that her thesis advisor, astronomer Henry Norris Russell, initially dismissed her findings as “impossible.” However, after verifying her data, he realized she was correct.
Payne was a gifted teacher, and many of her students went on to have successful careers in science. Her approach to teaching was based on hands-on experimentation and observation, which helped to instill a deep understanding of the natural world in her students.
Payne was an advocate for women in science and worked tirelessly to promote their participation in the field. She mentored numerous women scientists throughout her career and was a vocal critic of the gender-based discrimination she faced.
In addition to her scientific work, Payne was also a talented musician and played the violin. She often played in orchestras and chamber groups, seeing music as a way to balance out the intensity of her scientific work.
Overall, Cecilia Payne was a fascinating and multi-talented individual who made important contributions to both science and society. Her legacy continues to inspire and influence new generations of scientists, and her life serves as a reminder of the importance of perseverance, dedication, and the pursuit of knowledge.
There are, however, a few pieces of information about Cecilia Payne’s life and career that have been exaggerated or misunderstood over the years. These include:
- Payne did not “discover” the composition of the sun. While she was the first scientist to propose that the sun and other stars were mostly composed of hydrogen and helium, her findings built on the work of other scientists who had already established the basics of stellar spectroscopy. Additionally, other scientists, such as Henry Norris Russell, played a significant role in developing and refining the theory of stellar composition.
- Payne did not face as much discrimination and sexism as some later accounts suggest. While she did face some obstacles as a woman in a male-dominated field, such as being paid less than her male colleagues, she was also respected and admired by many of her peers. She had a close working relationship with Henry Norris Russell, who supported her research and helped to promote her career. Additionally, Payne herself did not view her gender as a major barrier to her success.
- Payne did not win the Nobel Prize for her work on stellar composition. While her discovery was groundbreaking, the Nobel Committee did not recognize the significance of her work until decades later. In fact, Payne was never awarded a Nobel Prize for her scientific contributions, although she did receive numerous other honors and awards throughout her career.
While these points may diminish some of the myths and legends that have grown up around Cecilia Payne, they do not detract from her many genuine accomplishments as a scientist and a trailblazer for women in science. Payne remains an important figure in the history of astrophysics and a role model for anyone who seeks to pursue knowledge and discovery.
According to her obituary, near the end of her life, Cecilia Payne-Gaposchkin wrote:
“Young people, especially young women, often ask me for advice. Here it is, valeat quantum:
Do not undertake a scientific career in quest of fame or money. There are easier and better ways to reach them. Undertake it only if nothing else will satisfy you; for nothing else is probably what you will receive. Your reward will be the widening of the horizon as you climb. And if you achieve that reward you will ask no other.”
Thanks and attribution:
https://www.harvardmagazine.com/2020/05/feature-vita-cecila-payne-gaposchkin
https://en.wikipedia.org/wiki/Cecilia_Payne-Gaposchkin
https://now.northropgrumman.com/cecilia-payne-gaposchkin-discovered-what-stars-are-made-of/
https://jtg.sjrdesign.net/advanced/sun_astar.html
https://www.snopes.com/news/2019/08/23/cecilia-payne-harvard-astronomy/
https://www.quora.com/How-can-you-describe-the-composition-of-the-Sun
https://www.famousscientists.org/cecilia-payne-gaposchkin/
https://www.britannica.com/biography/Cecilia-Payne-Gaposchkin
March 2023
Interesting Fact of the Month: Pioneers of Astronomy
As long as people have gazed up into the heavens, they have wondered about, and sought to explain, what they were seeing. From ancient times, philosophers, priests, scholars, and the merely curious have tried to put logic and order into the movements they observed and relate them to natural phenomena such as rain drought, seasons, and tides. Calendars and time itself were often set to observations of the sun and moon. The first evidence of recognition that astronomical phenomena are periodic and of the application of mathematics to their prediction is Babylonian, but India, Greece, Eqypt, China, and Mesoamerica all contributed to the understanding of astronomical observations.
Egyptian-born Ptolemy (Claudius Ptolemaeus) ca. 90 – ca. 168 C.E.), is most remembered for his development of the Geocentric (Earth-centered) cosmological system, known as the Ptolemaic model or cosmology that prevailed up until the 1500’s, and which is recognized as one of the most influential and longest-lasting, intellectual-scientific achievements in human history.
In Ptolemy’s cosmology, the Earth occupied the center of the universe, while other heavenly bodies lay beyond it in the following order: the Moon, Mercury, Venus, Sun, Mars, Jupiter, and Saturn—in his day, only five planets were known to exist. To account for the movements of these bodies, the Ptolemaic system used large circles centered on the Earth, along with smaller circles, or epicycles, that moved around the circumferences of the larger circles.
Ptolemy gave various reasons why the Earth must be both at the center of the universe and immovable. One argument was that because all bodies fall to the center of the universe, if the Earth were not at the center, things would not fall to the Earth, but the Earth itself would fall. He also argued that if the Earth moved, then things thrown vertically upward would not fall to the place from which they had been thrown, as they were observed to do. Scientists now recognize that all those reasons are false or mistaken.
So despite their apparent success in bringing order to man’s understanding of the universe, Ptolomy’s ideas were still theoretical and contained flaws that increasingly conflicted with observations and calculations made by observers. As it turned out, his computational methods were of sufficient accuracy to satisfy the needs of astronomers, astrologers, and navigators, until the time of the great explorations.
In the year 1492, the discovery of the New World initiated a frenzy of excitement for scientists who now knew the world was round instead of flat, leading Polish born Nicolaus Copernicus to suggest a heliocentric model of the universe which challenged the widely held belief that the Earth was at rest in the center of the universe, with the sun and other planets revolving around it. Considered one of the most significant astronomical thinkers of all time, Copernicus forever changed the way scientists understand the design of our solar system
His challenge to Ptolomy’s ideas was based on his conclusion that :
“ . . . the planetary theories of Ptolemy and most other astronomers, although consistent with the numerical data, seemed likewise to present no small difficulty. For these theories were not adequate unless certain equants were also conceived; it then appeared that a planet moved with uniform velocity neither on its deferent (main orbit) nor about the center of its epicycle (second orbit) … Having become aware of these defects, I often considered whether there could perhaps be found a more reasonable arrangement of circles, from which every apparent inequality would be derived and in which everything would move uniformly about its proper center, as the rule of absolute motion requires.”
The proposition of Copernicus’ cosmological beliefs sparked controversy in both scientific and religious communities, and his ideas were generally disregarded. However, within roughly one hundred and fifty years, the work of other planetary scholars such as Kepler, Galileo, and Newton eradicated any disbelief and put Copernicus’ original ideas into fruition. In this way the Copernican Revolution can be considered the beginning of modern astronomy.
Other figures also aided this new model despite not believing the overall theory, like Danish-born Tycho Brahe, with his well-known observations. Brahe created the Tychonic system, where the Sun and Moon and the stars revolve around the Earth, but the other five planets revolve around the Sun.
This system blended the mathematical benefits of the Copernican system with the “physical benefits” of the Ptolemaic system. This was one of the systems people believed in when they did not accept heliocentrism, but could no longer accept the Ptolemaic system. He is most known for his highly accurate observations of the stars and the solar system.
Brahe was assisted by Johannes Kepler, who would later use his observations to finish Brahe’s works and for his theories as well. After the death of Brahe, Kepler was deemed his successor and was given the job of completing Brahe’s uncompleted works. Like many other figures of this era, he was subject to religious and political troubles. Kepler was, however, the first to attempt to derive mathematical predictions of celestial motions from assumed physical causes. He discovered three laws of planetary motion that now carry his name, those laws being as follows:
1.The orbit of a planet is an ellipse with the Sun at one of the two foci.
2. A line segment joining a planet and the Sun sweeps out equal areas during equal intervals of time.
3. The square of the orbital period of a planet is proportional to the cube of the semi -major axis of its orbit.
With these laws, he managed to improve upon the existing heliocentric model, giving it more credibility because it adequately explained events and could cause more reliable predictions. Before this, the Copernican model was just as unreliable as the Ptolemaic model. This improvement came because Kepler realized the orbits were not perfect circles, but ellipses.
Italian Galileo Galilei’s observations through a self-built telescope strengthened his belief in Copernicus’ theory that Earth and all other planets revolve around the Sun, as they in fact do, but it woefully failed to grasp the magnitude of the heavens these pioneer astronomers were observing.
As recently as 1923, the universe was still thought to be a small place. Astronomers believed the Milky Way galaxy was at least tens of thousands light-years across, but they thought it was all there was to the universe — one grand galaxy spiraling in a lonesome ballet.
To be sure, the Milky Way is an enormous place, with at least a hundred billion stars. But American Edwin Hubble suspected there was so much more. Hubble, was perplexed by Andromeda, a fuzzy spiral region in the night sky.
At the time, the prevailing view was that Andromeda was located inside our own Milky Way. It was assumed to be a nebula, a region of gaseous space where stars are made.
Hubble was joined by a small but growing group of scientists — led by Heber Curtis of the Lick Observatory at the University of California — who were doubtful of this conclusion. To them, Andromeda was incredibly odd. A large number of supernovae — exploding stars — emanated from it, many more than you’d expect from a similarly sized swath of the night’s sky. What’s more, these numerous supernovae were all very faint, as if they were very far away. Hubble and Curtis hypothesized Andromeda was its own “island universe,” a self-contained system of stars, much like our own Milky Way. At the time, this was an outrageous idea, and was met with stiff, stubborn resistance by the scientific establishment. It would mean the universe was at least double its imagined size.
In 1920, Harlow Shapley, an astronomer who had just produced the most accurate measurement of the Milky Way to date, engaged Curtis in a fierce “great debate” at the National Academy of Sciences. Shapley held the view that the Milky Way and the Universe were one and the same, and Andromeda was a “truly nebulous object.”
More evidence was needed for either argument. And Hubble was the one to find it.
To prove Andromeda existed outside the Milky Way, Hubble would need to measure how far away it was from Earth. If it was farther away than the estimated diameter of the Milky Way, then it couldn’t be inside the Milky Way. Hubble’s work here stands on the back of another great but less well-known astronomer, a woman named Henrietta Leavitt. Leavitt was an astronomer at the Harvard College Observatory in the early 1900s, where she was employed as a “computer,” a person whose job it was to support the senior astronomers by making mathematical calculations (much like digital computers do today). In 1908, while making calculations of a class of stars called cepheids, she made a wild discovery.
Cepheids are stars that periodically dim and brighten. Leavitt discovered that the time it took for these stars to cycle could be used to calculate how far away the stars are in the sky. With this discovery, astronomers finally had a yardstick, a way to measure the distance to objects in the cosmos. (Learn more about Leavitt here.)
All Hubble had to do was look for cepheid stars in Andromeda and make the appropriate calculations. Night after night, he took photographs of Andromeda with the enormous telescope, searching for cepheids. In October 1923, he found one, blinking in one of Andromeda’s spiral arms. A week more of observations allowed him to follow Leavitt’s formula and determine its distance.
The light from V1 made it clear: Andromeda was well outside the bounds of the Milky Way. When Shapley received a letter about Hubble’s results, he reportedly said, “Here is the letter that has destroyed my universe.”
Destroyed it, yes. But its shattering also made it larger. The Milky Way was no longer the entire universe. It was more like one tiny grain of sand on a beach; a ballet with an untold number of dancers.
Today, the universe keeps growing larger. Astronomers now believe there are some 2 trillion galaxies stretching out over 90 billion light-years. Wherever we look in the cosmos, there are endless mysteries to uncover. Hubble taught us that these mysteries can be bigger and grander than we ever imagined.
As Hubble said in 1948: “Equipped with his five senses, man explores the universe around him and calls the adventure Science.”
Thanks and attribution:
https://en.wikipedia.org/wiki/History_of_astronomy
https://www.newworldencyclopedia.org/entry/Ptolemy
https://discover.hubpages.com/education/Scientifis-Revolutions-Nicolaus-Copernicus
https://greatestminds.net/nicolaus-copernicus-things-you-may-not-know/
Repcheck, Jack. Copernicus’ Secret: How the Scientific Revolution Began (New York, Simon & Schuster, 2007), 54
https://gohighbrow.com/wp-content/uploads/2015/03/78.jpg
https://starchild.gsfc.nasa.gov/docs/StarChild/whos_who_level2/galileo.html
https://www.patrickarundell.com/World-News-Astrology/wp-content/uploads/2019/02/33-48.jpg
https://www.nasa.gov/centers/goddard/news/topstory/2004/0107filament.html
https://www.vox.com/2016/11/20/13677046/edwin-hubble-andromeda-galaxy
https://www.britannica.com/biography/Edwin-Hubble
https://www.famousscientists.org/henrietta-swan-leavitt/
February 2023
Interesting Fact: NASA Spinoffs Make Our Lives Better
Since NASA technology is taxpayer funded, it is shared with the American people and by extension, the people of the world. New technologies shared in this way are called Spinoff’s and the public has benefitted from over 2,000 of them since 1976, often in the form of commercial products. Some, such as Tang and Teflon, are widely known but many are not. Herewith is a short list of some of the more interesting and possibly surprising ways in which NASA engineering and research have become part of our lives, helping to solve some of our most pressing societal and environmental challenges.
1. Lightning in a Bottle.
From 1997 to 2017, the National Space Biomedical Research Institute (NSBRI) looked into how long-duration spaceflight affected people, funded with grants from NASA’s Johnson Space Center in Houston. One area of interest was light sources and how they affected brain activity. The NSBRI funded two professors, Thomas Jefferson University’s George Brainard and Harvard Medical School’s Steven Lockley, to learn more. Lockley and Brainard’s research found that exposure to excess blue light at the wrong time could throw off a person’s body. This happened because the body’s production of melatonin, a key hormone for managing circadian rhythms, was inhibited when certain wavelengths of blue light hit photoreceptors in the eyes.
When the fluorescent lighting fixtures on the space station needed to be replaced in 2011, NASA looked to the growing field of solid-state light-emitting diodes (LEDs). Solid-state means that there’s no physical reaction creating light, unlike an incandescent bulb or fluorescent plasma. In creating a new lighting system, the findings of the NSBRI’s research played a big role.
“When NASA started looking into [LED fixtures], the industry didn’t even have standards for solid-state lighting. They didn’t have any information, certainly not a lot of research on large-scale implementation of circadian lighting,” said Toni Clark, testing lead for spacecraft lighting at Johnson.
Researchers there and engineers at NASA’s Kennedy Space Center in Florida worked together on the Solid-State Lighting Assembly (SSLA) for the space station. The lamp module was primarily built by Bionetics Corporation, which also had labs and manufacturing facilities on-site at Kennedy. The new lighting modules, installed in 2016, consumed far less energy and emitted far less heat than previous fluorescent lighting on the station. In addition, they changed color, temperature, and intensity in a 24-hour cycle, in accordance with the NSBRI’s circadian lighting research.
The change paid off, as astronauts reported feeling better-rested. LED modules also helped in plant-growth experiments on the space station, with the lights providing the right wavelengths for photosynthesis.
2. Keeping Warmer in the Great Outdoors:
Not everyone would celebrate the new year camping out in the Rocky Mountains during some of the longest, coldest nights of the winter. But that’s how Jon Rosenberg and his wife rang in 2018, and they learned the hard way that the outdoor gear market still didn’t entirely take such extreme conditions into account. In just one night, the cold killed the batteries in the camera his wife, a professional photographer, brought with them.
“Other insulated products will keep groceries cold for a week, but if all you need is to keep batteries or a snack from freezing, there’s not much of a solution,” he said. Rosenberg, who worked in the outdoor gear industry, knew of a class of high-performance insulation pioneered by NASA to keep cryogenic rocket fuel chilled to minus 423 degrees Fahrenheit in the balmy heat of Florida. The key to these insulators is a substance called aerogel.
The first aerogels were invented before NASA even existed. They’re made by removing all the moisture from a gel – usually made of silica – while leaving the solid structure intact. The resulting material is almost entirely air, pocketed in tiny chambers. It weighs next to nothing and is nearly impervious to heat. However, until NASA got involved, it was also too fragile to use.
In the 1990s, NASA’s Kennedy Space Center in Florida was looking for better insulation to maintain the frigid temperatures needed for storing space shuttle fuel.
The best cryogenic insulations at the time were layered metallized thin films, invented by NASA in the early days of the space program. These reflected radiated heat and could be placed in a vacuum to prevent heat conduction or convection. But they were relatively expensive and heavy, and their ability to conduct heat along their surfaces caused complications.
A company called Aspen Systems, however, had an idea for incorporating aerogel into flexible insulating blankets. With Small Business Innovation Research (SBIR) funding from Kennedy, and then from a handful of other NASA field centers, Aspen developed a process to coat insulation fibers with aerogel to trap air while also stopping heat conduction.
First, Aspen treated the fibers, both to make them reflective and to help them bond with an aerogel. Then it soaked them in a liquid aerogel precursor material and flash-dried them at high temperature and pressure to turn the liquid to solid aerogel. Every fiber in the resulting blankets was enveloped in air-filled aerogel, preventing the fibers from touching each other to conduct heat. Moreover, the air molecules trapped in the aerogel’s countless microscopic cells also couldn’t touch each other to transfer heat, and they couldn’t move across the insulation or even escape when it was squeezed or soaked.
Today the space agency uses aerogel in myriad ways, and Aspen Aerogels has spun off to specialize in what became one of the most successful, widespread spinoffs in NASA’s history, incorporating aerogel into a host of outdoor wear, building and industrial insulations, and more. And like many of the agency’s most successful inventions, aerogel-infused insulation has spun off well beyond NASA, spawning partnerships and product lines that are two or more degrees removed from the original work but still owe their existence to NASA’s need-driven investment.
One company that’s recently entered the aerogel market is Latham, New York-based PrimaLoft, a brand known for high-performance insulation since the 1980s. After a group of PrimaLoft employees toured the Aspen Aerogels manufacturing facility and became inspired, the company began developing two new lines of aerogel insulations. Today that decision is paying dividends in bulk: scores of brands are already using this newest spin on NASA’s early forays into aerogel to make warmer outdoor gear.
3. Space Age Water Conservation:
The more difficult a problem is for NASA, the more solutions it eventually produces for the rest of us. Few challenges are more pressing for the space agency than the need for clean water. Water is heavy — much heavier than the liquid hydrogen and oxygen NASA uses for rocket fuel — and every pound launched into space costs thousands of dollars. So on the space station, nothing is wasted — sweat, urine, and even breath moisture are collected, purified, and recycled as drinking water. But despite its origins, the water astronauts drink is cleaner than what’s available to most people on Earth.
To achieve this, NASA has pushed the cutting edge of water purification since the agency’s early years. And most of these innovations have found plenty of use here on the ground, too, in homes and water bottles, in industrial settings, and in remote locations where safe drinking water is scarce. As the worldwide demand for fresh water grows, this technology becomes more essential every day, as it ensures that people have enough safe water to drink, treats polluted water, and eases the demand on natural aquifers.
These are just a few examples of NASA technology now cleaning water on Earth.
In one recent development, an unlikely partnership between NASA and a Swedish university — with the help of filter technology the space agency helped develop almost 20 years ago — led to the world’s first water-recycling shower. In 2012, Mehrdad Mahdjoubi, then a master’s student in industrial design at Lund University in Sweden, traveled to Johnson Space Center as part of an annual program to learn about the challenges of designing habitats for astronauts. The focus was on a five-year Mars stay. Current astronauts, short on water and gravity, take sponge baths, but Mahdjoubi thought spacefarers with feet planted on Martian ground would prefer a real shower. But water on Mars’ desert surface is still scarce, so Mahdjoubi came up with an idea for reusing the flow. “I’d have never thought about doing something like this if I didn’t have that NASA experience,” he says.
To rapidly purify and reuse water, he hit on an especially thorough water filtration technology developed in part with NASA funding, known as NanoCeram. Other filters have micropores tiny enough to physically filter out bacteria and even viruses, but these are painfully slow. A material invented by Argonide Corporation, however, made up of positively charged microscopic alumina fibers, can remove virtually all contaminants, including bacteria and viruses, despite having significantly larger pores — allowing a much higher flow rate. The positive charge of the fibers attracts and traps microorganisms and other contaminants, which generally carry a negative charge. Activated carbon in the filter aids in snaring particulate, chemical, and soluble contaminants.
Under two Small Business Innovation Research contracts from Johnson in the early 2000s, Argonide optimized its nanofibers for strength and virus adhesion and built, tested, and validated full-scale filter models. Since then, other companies have used the filters in water bottles, portable humanitarian units, and industrial water purification. And now Mahdjoubi has incorporated the filter into a recirculating shower known as Oas — Swedish for “oasis” — built and marketed by his new company, Orbital Systems.
The shower starts with less than a gallon of water and circulates it at a rate of three to four gallons per minute, more flow than most conventional showers provide. The system checks water quality 20 times per second, and the most highly polluted water, such as shampoo rinse, is jettisoned and replaced. The rest goes through the NanoCeram filter and then is bombarded with ultraviolet light before being recirculated. The Swedish Institute for Communicable Disease Control has verified that the recycled water is cleaner than tap water. And because the reused water is already warm, it takes minimal energy to heat it back to the target temperature.
“There’s a general assumption you can’t do anything about water conservation without compromising your life quality,” says Mahdjoubi. “But we don’t tell people to stop showering, and we don’t destroy their experience. We enhance it with a higher flow rate. The ability to save without sacrifice, I think, is the most important part of our value proposition.”
Orbital Systems, headquartered in Sweden with U.S. offices in Sausalito, California, has raised about $50 million in investments and employs 60 to 70 people. The company has sold several thousand units, so far mostly to hotel chains and real estate developers, and the showers are becoming available to individual consumers in Sweden, Denmark, and Germany this year. Mahdjoubi plans to expand to North America, Asia, and beyond but first needs to enlist distributors and certify installers. In the longer term, he wants to enable an entire habitat that runs on closed loops, recycling as much and using as little water as possible, just as a Martian habitat would.
“As humans, we’re really good at innovating, but we tend to be quite complacent until we actually have to do it,” Mahdjoubi says. “Designing for Mars forces more creativity.”
4. Using Nature’s R&D Lab
One of the most remarkable — and possibly most effective — water-treatment technologies NASA has explored is a membrane embedded with the same natural proteins that transport water through the membranes of living cells. Known as aquaporins, these proteins are what allow plant roots to absorb water from soil and human kidneys to filter about 45 gallons of blood per day. They can transport water through cell membranes one molecule at a time, while rejecting other substances.
Now companies all over the world are looking at various ways these membranes can be used, including many pilot wastewater treatment projects, whether adding efficiency to existing wastewater treatment facilities or purifying wastewater that until now has gone untreated, polluting groundwater and waterways.
The concept has been pioneered by Danish company Aquaporin A/S, and when Ames Research Center learned what the company was trying to do in 2007, the center became the first paying customer. Ames and the European Space Agency tested the membranes on flights to the International Space Station.
Astronauts on the ISS tested prototype water purification systems based on membranes infused with aquaporin proteins. The testing, which validated both forward and reverse osmosis-based systems, was sponsored by the European Space Agency and overseen by NASA. Image courtesy of the European Space Agency
These operate through reverse osmosis, using pressure to push water through the filters. But the company had even bigger plans for the membranes in forward osmosis, a process that drives itself without any outside influence. With saltwater on one side of the membrane and wastewater on the other, thermodynamics compel the salt to distribute itself evenly throughout all the water in the system. But because salt can’t pass through the membrane, it draws the fresh water through from the other side, leaving only waste.
And in healthcare, forward osmosis has the potential to make dialysis portable, improving patients’ quality of life, he says. Dialysis patients often spend hours traveling to and from treatment three or four times a week, where they spend another three or four hours bedridden, creating a sedentary lifestyle. This is partly because hemodialysis a massive water consumer. The process requires about 200 liters of water per treatment—all of which ends up as wastewater. If clean water were extracted from the waste and reused throughout the treatment, he says, dialysis could fit in a backpack.
“We’re testing forward osmosis in a closed water-recycling loop with a lot of players in dialysis,” Holme Jensen says. “Medical device companies understand and pick up on the technology because if you look at how a kidney works, it reuses water in a closed loop.”
And after all, the mechanism that makes that closed loop work is the aquaporin protein.
5. Making GPS more accurate:
In the 1960s, NASA used a network of radio telescopes and a technique called very large baseline interferometry (VLBI) to capture images of quasars in distant galaxies. In the following decade, scientists reversed the process to determine the precise locations of the telescopes, painting a picture of Earth’s shape and orientation in space. Today, an evolution of that technology supports another location-based system that has arguably become the world’s most important communication infrastructure: the Global Positioning System (GPS).
When the first GPS satellite was launched in 1978, NASA’s Jet Propulsion Laboratory (JPL) already had experience in tracking radio signals from faraway sources and extracting valuable information from them. Using VLBI, for example, JPL scientists measured the time difference between when the same radio signals from quasars—some of the brightest objects in the universe—hit different telescopes around the world to conduct geodesy, assessing Earth’s size and shape.
These capabilities served as the springboard that let JPL recognize early on the potential that GPS held and compelled the center to invest in the technology and infrastructure to support it.
In the early 1980s, as the U.S. Air Force continued launching GPS satellites, JPL started building out a tracking network, collocating the first ground stations with VLBI tracking sites. These offered the necessary communications infrastructure and precise location information to tie the geodetic reference frame to that of GPS. Today the network includes more than 80 receivers, making it the world’s largest geodetic-quality, centrally managed GPS tracking network.
Raw GPS data can produce positioning errors of 30 feet or more if not calibrated for signal delays caused by electrons and gases in Earth’s atmosphere, errors in GPS satellite positions, and noise and drift in the satellites’ atomic clocks. The software JPL developed to model delays, correct for errors, and perform GPS orbit determination and receiver positioning was called GIPSY-OASIS (GPS-Inferred Positioning System and Orbit Analysis Simulation Software). It quickly became one of NASA’s most widely licensed software programs, with hundreds of licenses to academia and industry.
But no one was able to make these corrections in real time on a global scale until the Internet became widespread in the mid-1990s. Shortly after the GPS satellite fleet became fully operational in 1994, JPL received a small amount of funding from NASA’s Deep Space Network program to figure out how to use GPS data in real time to enable faster turnaround time for deep-space navigation. That seeded the development of JPL’s Real-Time GIPSY (RTG) software, which could make all these corrections and update them every second.
The corrections also were globally and uniformly valid, in contrast to the prevailing approaches at the time, which corrected signals based on local information, leading to corrections that were only valid regionally and suffered from degradation when the satellites rose and set on the target region.
Once JPL had demonstrated precision global GPS in real time, a host of new possibilities opened up.
“We took an innovative approach to the opportunities and challenges the free Internet offered, and we also had the vision to recognize that real-time GPS processing on a global scale could be revolutionary,” says Yoaz Bar-Sever, who was one of the developers of GIPSY and RTG and is now the manager of the Global Differential GPS (GDGPS) system at JPL. “But we were still surprised by the scope of the impact of the new capabilities we were building.”
In the mid-1990s, both industry and government began forays into the use of GPS corrections to improve positioning, navigation, and timing. In 1995, Satloc, a small company vying for the U.S. market of industrial GPS corrections, licensed RTG and became the first provider of RTG-corrected GPS service across most of North America.
The following year, the Federal Aviation Administration (FAA) selected the software as the prototype for its Wide Area Augmentation System (WAAS), with the goal of providing accurate GPS navigation for pilots, and offered funding for JPL to further mature the technology.
“We had the track record, and we had the only proven software to do what they wanted,” says Bar-Sever.
Another key investment in 2000 from the NASA Earth Science Technology Office helped JPL put in place the operational technology and infrastructure needed to launch a reliable, global, real-time service, and the GDGPS system was born. “As the Internet became more prevalent, we were able to get orbital determination in seconds globally,” Bar-Sever says. “That led a lot of industry to us.”
While NASA remained a key user of GDGPS, its ongoing development ever since has been funded almost entirely by other government users and commercial companies.
As word spread of the accuracy and global coverage of GDGPS, in 2002, the Air Force sponsored the development of a dedicated service to provide a wealth of information to its GPS operators, a service that has been enhanced over the years to adapt to the evolving GPS constellation and continues to this day. “In supporting operational GPS, the GDGPS system contributes to the reliability of the entire GPS enterprise,” says Bar-Sever.
Also in the early 2000s, GDGPS found its first major commercial customer in the farm equipment company John Deere, which invested heavily to help JPL engineers make the system practical and reliable for guiding self-driving tractors (Spinoff 2017). The technology made precision farming commonplace around the world. By this time, the system enabled positioning accuracy to within less than three inches.
These days, GDGPS also monitors and processes data from the world’s other navigation satellite systems—the Russian Global Navigation Satellite System, the Chinese BeiDou system, the Japanese Quasi-Zenith Satellite System, and the European Galileo system that’s expected to go into full operation this year. It’s an arrangement that benefits all users. “When you have more satellites, you can get more accurate and reliable positioning, especially in urban canyons,” Bar-Sever says. “It also improves atmospheric observation. It enhances the overall capability.”
The JPL team is working, for example, to achieve unprecedented accuracy to guide self-driving cars. “The accuracy that industry demands is very difficult to achieve,” Bar-Sever says. “We’re working on that with the support of the aggregate of our customers.”
5. Helping to Feed the World. A New Generation of Indoor Farming
The first vertical farm in the U.S. provided a foundation for expanding the controlled environment agriculture industry
Originally published 11/23/2021 The United Nations predicts Earth will have to feed another 2.3 billion people by the year 2050, with most concentrated in urban centers far from farmland. Current agriculture may not have the capacity to feed that many people, and it could threaten future production by depleting soil of essential nutrients and contaminating freshwater supplies and soil with pesticides and herbicides. Conventional farmers are working to make their fields more efficient and productive and less harmful to the environment – at times with innovations and other support from NASA – but these changes are only part of a long-term solution.
NASA has been working for decades to tackle similar problems for space exploration. Reusing a limited water supply, minimizing energy consumption, and eliminating soil as a growth medium are just a few ways the agency stretches the limited resources available in space. Research into solving these challenges to grow plants in a closed environment like a spacecraft inspired NASA to build the first vertical farm in the United States, creating a foundation for the controlled environment agriculture industry to build on. The lessons learned are inspiring an unconventional new generation of farmers.
Called controlled environment agriculture or CEA, this combination of plant science and environmental control techniques optimizes plant growth inside an enclosed space. Unlike a traditional greenhouse, this new vertical approach to cultivation leverages technology and data to maintain ideal growing conditions in a completely closed structure. These tools make it possible to filter contaminants from water for crops (keeping them out of food) and deliver the exact nutrient balance to feed any crop throughout its life cycle. Artificial lighting can eliminate the effects of fluctuating solar light, nurturing growth with a precise mix of beneficial red, blue, and green light at the right intensity and duration. Environmental controls also maintain proper temperature and humidity to prevent disease.
These practices could help feed Earth’s burgeoning future generations, said Nate Storey, chief science officer at Plenty Unlimited Inc., one of several companies building on NASA plant-growth research with an eye toward bringing agriculture into the urban environment.
Thanks and Attribution:
https://spinoff.nasa.gov/lighting-in-a-bottle
https://spinoff.nasa.gov/Spinoff2019/ps_5.html
https://spinoff.nasa.gov/Spinoff2019/ps_1.html
Mike DiCicco Managing Editor (Using Nature’s R&D Lab)
January 2023
Interesting Fact: Preparing to Explore Mars
If you are planning a trip, especially a long one, you generally and prudently make preparations. For a trip across the country you would at least check airline schedules and weather forecasts in order to choose the right time to travel and the right clothes to pack. A foreign trip would probably mean researching entry requirements, vaccinations, COVID-19 restrictions, and making sure your passport is valid even beyond the end of your trip, in case of delays or other complications. You would investigate lodging availability and make reservations for side trips or other opportunities in the vicinity of your main destination. If your trip involved hunting, fishing, or mountain climbing, etc., you would pack the requisite equipment needed for a successful outcome.
But what would you do if your destination was somewhere that nobody else had ever visited? Someplace where little was known about conditions and where a mistake in your preparations could easily be fatal? What if your destination was Mars? What would you do to prepare for that? What could you do?
Fortunately, preparations have been underway for quite a while and much has been learned that increases the likelihood of a successful exploratory venture. From an engineering standpoint we have learned much from the Apollo, Space Shuttle, and ISS missions that can be applied to a Mars mission. However Mars, on average, is 140 million miles or more than 143 times farther away from the earth than is the moon. So instead of a 4 day trip to the moon, the time taken to travel the average distance of 140 million miles from Earth to Mars would be about 8.4 months each way. Researchers have been at work for decades to develop the means to accomplish the travel portion of such a trip.
However, what about preparations for actually being there? After all, that would be the point of undertaking such a large and expensive trip. To make the most of such a visit scientific instruments have already been sent there, returning data on conditions we can expect to experience. Since 1960 spacecraft have been sent to flyby, orbit, and, more recently, land and explore the surface of the planet.
The U.S. Mariner 4, launched on November 24, 1964, was the fourth in a series of spacecraft used for planetary exploration in a flyby mode and represented the first successful flyby of the planet Mars, returning the first pictures of the Martian surface. These represented the first images of another planet ever returned from deep space. Mariner 4 was designed to conduct closeup scientific observations of Mars and to transmit these observations to Earth. Other mission objectives were to perform field and particle measurements in interplanetary space in the vicinity of Mars and to provide experience in and knowledge of the engineering capabilities for interplanetary flights of long duration.
The Russian Mars 2 and Mars 3 missions, launched in 1971, consisted of identical spacecraft, each with a bus/orbiter module and an attached descent/lander module. The primary scientific objectives of the Mars 2 orbiter were to image the Martian surface and clouds, determine the temperature on Mars, study the topography, composition and physical properties of the surface, measure properties of the atmosphere, monitor the solar wind and the interplanetary and Martian magnetic fields, and act as a communications relay to send signals from the lander to Earth.
The Mars 2 attempted landing failed most likely because its parachute system didn’t deploy.
Through aerodynamic braking, parachutes, and retro-rockets, the Mars 3 lander did achieve a soft landing and began operations. However, after just 20 seconds the instruments stopped working, perhaps as a result of the massive surface dust storms raging at the time of landing.
The Viking 1 and 2 missions in 1976 were the first scientifically successful landers. The primary mission objectives were to obtain high resolution images of the Martian surface, characterize the structure and composition of the atmosphere and surface, and search for evidence of life. The results from the Viking experiments gave our most complete view of Mars. Volcanoes, lava plains, immense canyons, cratered areas, wind-formed features, and evidence of surface water are apparent in the Orbiter images.
Subsequent missions such as Mars Pathfinder, Mars Express (including the Spirit and Opportunity rovers), the Mars Science Laboratory (aka the Curiosity Rover), and the recent Mars 2020 mission, including the Mars Rover (Perseverance) and Hovercraft (Ingenuity), have revealed much information on the conditions and environment that will be faced by the first human explorers of Mars. The primary science objectives of Mars 2020 are to identify past environments capable of supporting microbial life, seeking signs of possible past microbial life, collecting core rock and regolith samples and caching them on the surface for future missions, and testing oxygen production from the Martian atmosphere.
While all these robotic explorations have been going on, human researchers have performed field research in areas identified as “Mars Analogs”. These are places on earth that bear some resemblance to the Martian environment as understood from the data received from the earlier instrumented missions.
Some examples of analog tests with people include NASA conducting a 120-day study in Hawaii to test a space food diet (HI-SEAS), and equipment tests inside Austrian mountain caves in 2012. A future Mars base has been compared to the Amundsen-Scott South Pole Station in Antarctica, because relatively small groups must survive in extreme conditions there.
Mars analogs are sometimes chosen for their location, for example, Devon Island is at 75°N latitude which provides solar radiance similar to the Martian Equator. Similarly, high altitudes can provide an equivalent to the low pressure of the Mars atmosphere.
Others include:
Mars-500, simulated a 520-day mission in Moscow.
- Mars Analogue Research Station Program, simulated Mars habitats
The European Space Agency’s list of ‘planetary analogues’ includes projects in Chile, Peru, South Africa, Namibia, Morocco, Italy, Spain, Canada, Antarctica, Russia, China, Australia, India, Germany, Norway, Iceland, and nine U.S. states. Israeli scientists plan to run shorter simulations in a nature preserve called D Mars.
Pictured is Gernot Groemer, commander of the AMADEE-18 Mars simulation in the Dhofar desert of southern Oman. The desolate desert in southern Oman resembles Mars so much that more than 200 scientists from 25 nations organized by the Austrian Space Forum are using it for the next four weeks to field-test technology for a manned mission to Mars
- Arctic Mars Analog Svalbard Expedition, annual expeditions to remote sites
- Concordia Station, multi-purpose Arctic base
Concordia Research Station, which opened in 2005, is a French–Italian research facility that was built 3,233 m (10,607 ft) above sea level at a location called Dome C on the Antarctic Plateau, Antarctica.
Concordia Station shares many stressor characteristics similar to that of long-duration deep-space missions, in particular extreme isolation and confinement, and therefore serves as a useful analogue platform for research relevant to space medicine. During the winter, the crew are isolated from the outside world, having no transportation and limited communication[2] for 9 months and live a prolonged period in complete darkness, at an altitude almost equivalent to 4000m at the equator. This creates physiological and psychological strains on the crew. Concordia station is particularly useful for the study of chronic hypobaric hypoxia, stress secondary to confinement and isolation, circadian rhythm and sleep disruption, individual and group psychology, telemedicine, and astrobiology. Concordia station has been proposed as one of the real-life Earth-based analogues for long-duration deep-space missions.[3]
HI-SEAS, crewed tests in Hawaiian mountains.
Located approximately 8,200 feet above sea level, the HI-SEAS habitat is a 1,200 square foot dome located on a Mars-like site on the Mauna Loa volcano on Hawai‘i Island. HI-SEAS has been the home to five successful long-duration (4 to 12 month) NASA Mars simulation missions and tens of other analog space missions in collaboration with multiple space agencies, companies, and organizations worldwide.
- NEEMO, underwater base,
NASA’s NEEMO 15 expedition will simulate aspects of a mission to an asteroid. In this illustration, a configured rock wall can be seen near the underwater Aquarius laboratory. (NASA)
NEEMO expeditions take place at the National Oceanic and Atmospheric Administration’s Aquarius Underwater Laboratory, which rests more than 62 feet (19 meters) below the ocean’s surface, off the coast of Key Largo in the Florida Keys.
- D-MARS, Israeli mission,
The D-MARS Desert Mars Analog Ramon Station project of Israel’s Space Agency, Ministry of Science was held near Mitzpe Ramon.
The experiment was conducted to investigate fields relevant to a future mission to the Red Planet, including satellite communications, the psychological effects of isolation, radiation measurements and searching for life signs in soil.
The mock Mars mission took place in an area whose surroundings resemble the Martian environment in its geology, aridity, appearance and desolation, according to the ministry.
- Lunares Research Station, European habitat in Poland.
Solar54, Argentina Mars Analog Base project
Two important considerations in attempting to find conditions on earth comparable those on Mars are Pressure and Gravity.
Pressure
At about 28 miles (45 km, 150 thousand feet ) Earth altitude the pressure starts to be equivalent to Mars surface pressure. However, the major component of Mars air, CO2 gas, is denser than Earth air for a given pressure. Perhaps more significantly there is no land at this altitude on earth. The highest point on earth is the summit of Mount Everest at about 5.5 miles (8.8 km, 29 thousand feet), where the pressure is about fifty times greater than on the surface of Mars. The correct atmospheric pressure can be created by a vacuum chamber. NASA‘s Space Power Facility was used to test the airbag landing systems for the Mars Pathfinder and the Mars Exploration Rovers, Spirit and Opportunity, under simulated Mars atmospheric conditions.
Gravity
The gravity of Mars is about 38% of Earth’s gravity at the surface, about 3.7 meters per second. This can be simulated for short time by an aircraft following a flight profile that causes this type of acceleration. This technique (using a variation on free-fall) has allowed the gait of people in Mars gravity to be studied.
Chile’s Atacama Desert is the driest place on Earth — and a ready analog for Mars’ rugged, arid terrain. Compare this site with an image of Mars itself:
Many people will be surprised at the extent of the technological advances and research preparations that have already been made and must continue to be made in support of the first crewed mission to Mars. It is not clear if they will need passports, but what a visa stamp that would be!
Thanks and attribution:
https://treehozz.com/how-far-away-is-mars-compared-to-the-moon
https://www.distances-calculator.com/distance-planet-earth-to-mars.php
https://nssdc.gsfc.nasa.gov/nmc/spacecraft/display.action?id=1964-077A
https://nssdc.gsfc.nasa.gov/nmc/spacecraft/display.action?id=1971-049A
https://nssdc.gsfc.nasa.gov/planetary/viking.html
https://nssdc.gsfc.nasa.gov/nmc/spacecraft/display.action?id=2020-052A
https://en.wikipedia.org/wiki/List_of_Mars_analogs
https://mars.nasa.gov/resources/8351/atacama-landscape/
https://en.wikipedia.org/wiki/Concordia_Station
https://www.sciencephoto.com/media/730925/view/mariner-4-and-mars