6.0 Natural and Induced Environments
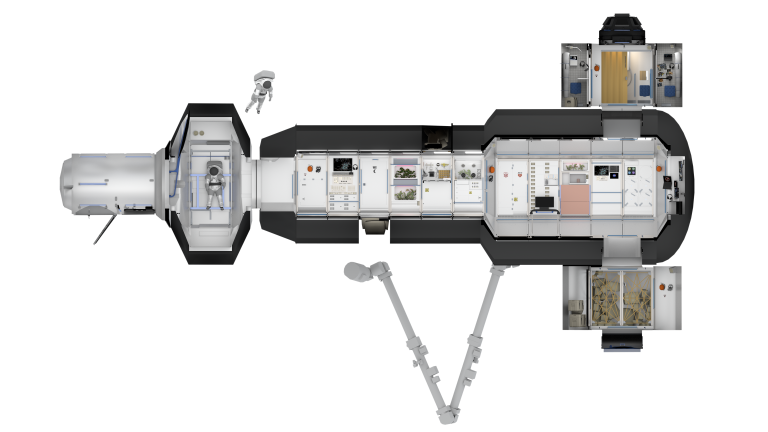
6.0 NATURAL AND INDUCED ENVIRONMENTS
Natural and induced environmental factors include air, water, contamination, acceleration, acoustics, vibration, radiation, and temperature. Environmental design factors that can enhance human performance such as crew station layout and lighting are discussed in section 8, Architecture, in this NASA Technical Standard. Overall, the system’s environment is to be compatible with tasks to be performed and promote crew health and performance.
6.1 Trend Analysis of Environmental and Suit Data
[V2 6001] The system shall provide environmental and suit monitoring data in formats compatible with performing temporal trend analyses.
[Rationale: Requirements are to consider all environmental and suited parameters that may require trend analysis for a given mission. Trending of environmental parameters such as internal atmosphere constituents, temperature, humidity, water, acoustics, radiation, acceleration and dynamic loads, suited biomedical data and suit data (i.e. suit temperature, suit humidity level, suit pressure, ppO2, ppCO2, metabolic rate and trends of these data points) (see sections 6 and 11 in this NASA Technical Standard for the detailed requirements) is necessary for both anticipating harmful conditions before they occur and troubleshooting using previously stored data. To properly trend, aspects of the data such as the measurement rate are also to be considered, as some parameters may otherwise only be measured infrequently.]
6.2 Internal Atmosphere
A safe, breathable atmosphere is critical to human health and performance. Early identification of potential air quality issues enables mitigation by design. Monitoring atmospheric quality and evaluating trends are essential. The system is to be robust enough to control or allow crew control of atmospheric pressure, humidity, temperature, ventilation flow rate, airborne particulates, partial pressure of O2 (ppO2), partial pressure of CO2 (ppCO2), and trace contaminants within ranges necessary to maintain task performance and human health and safety. Although the following requirements address these components individually, they must be considered as a whole because they are dependent on each other. Additional information about the interplay among internal atmosphere components and the effects on the human is available in section 6.2 of the Human Integration Design Handbook (HIDH). An iterative process should be employed to integrate the human system internal atmospheric limits into the design process, ensuring optimization of the design to afford the most protection possible, within other constraints of the vehicle systems. Atmospheric requirements specific to suited operations can be found in section 11.1, Suit Design and Operations, in this NASA Technical Standard.
6.2.1 Atmospheric Constituents
6.2.1.1 Inert Diluent Gas
[V2 6002] Cabin atmospheric composition shall contain at least 30% diluent gas (assuming balance oxygen).
[Rationale: This mitigates clinically significant absorption atelectasis. The choice of diluent gas is dependent on many factors, including physiological activity and contribution to decompression sickness (DCS). The assumption is that the diluent gas will be nitrogen (N2). Use of helium (He) or argon (Ar) has been considered, but would affect decompression sickness (DCS), thermal and ventilation requirements. Validation and verification of alternate diluent gases must be adjudicated by program medical team and another requirement set be developed. Measurable oxygen absorption atelectasis (lung collapse) occurs with fractions of oxygen above 50%. A diluent gas is required to prevent excessive levels of oxygen absorption atelectasis during prolonged exposures, in addition to reducing the ignition/flammability threshold. Unacceptable risk occurs when breathing less than 20% diluent. Although oxygen absorption atelectasis can be observed in the 25-50% diluent range, clinical and spaceflight experience shows that diluent fractions as low as 25% maintain acceptable levels of pulmonary function for long duration spaceflight activities. Clinically significant atelectasis is unlikely when combining the expected habitat total pressures (typically 8.2 to 14.7 psia) with the hyperoxic bounds established in Table 6.2-1—Inspired Oxygen Partial Pressure Exposure Ranges. Reference [V2 6003] O2 Partial Pressure Range for Crew Exposure in this NASA Technical Standard for hyperoxic/hypoxic limits.]
6.2.1.2 O2 Partial Pressure Range for Crew Exposure
[V2 6003] The system shall maintain inspired oxygen partial pressure (PIO2) in accordance with Table 6.2-1—Inspired Oxygen Partial Pressure Exposure Ranges.
[Rationale: For all systems, the range of ambient dry-gas ppO2 is to be considered in the context of PIO2. Since physiological limits for hypoxic (and hyperoxic) exposure during spaceflight are unknown, systems designed for humans are to be normoxic unless strong rationale is provided for an alternative suggestion. For confined and enclosed spaces at sea level, the Occupational Safety and Health Administration (OSHA) defines oxygen-deficient atmosphere as <19.5% oxygen by volume, and oxygen-enriched atmosphere as >23.5% oxygen by volume (Electronic Code of Federal Regulations, 1910.146(b)(3), current through July 29, 2021) with a correlating normoxic PIO2 range between 139-168 mmHg. The OSHA-enriched atmospheric limit is more applicable to material flammability than human hyperoxic limit. Hyperoxia limits are provided to allow needed prebreathe and if necessary, DCS treatment. It is generally accepted that there are no medical or performance issues with constant exposure to one-half an atmosphere of O2 partial pressure (Clarke, J.M., Oxygen Toxicity [Chapter 6]. The Physiology and Medicine of Diving [4th ed], Bennett, P.B., Elliott, D.H. [eds]. W.B. Saunders Company Ltd: Philadelphia, 1993, pp. 153-69); this is a ppO2 of 7.35 psia, or 380 mmHg, or as PIO2, it is 333 mmHg. NASA operates the extravehicular mobility unit (EMU) at 4.3 psia with 100% O2, so this is a ppO2 of 222 mmHg; or as PIO2, it would be 175 mmHg, and there are no performance or medical issues to date with this limit. A practical and defensible hyperoxic exposure limit would be a PIO2 of 333 mmHg with the assumption that adaptations to microgravity do not modify this terrestrial limit. As far as a hypoxic limit, it has been shown that a mildly hypoxic vehicle system atmosphere as part of a denitrogenation protocol to reduce the risk of DCS is advantageous during spaceflight (e.g., Space Shuttle 10.2 psia/26.5% O2), as well as recommended by the Exploration Atmospheres Working Group and endorsed by Human Exploration and Operations Mission Directorate (Exploration Atmospheres, HEOMD memo, February 2013). There is no indication on Earth that living and working with chronic PIO2 of 127 mmHg degrades health or performance. There are no indications or predictions based on limited past experience that extending exposure time with PIO2 of 127 mmHg in micro or partial gravity past 7 days leads to degradation of health or performance in otherwise healthy astronauts. There is no opportunity to collect data in microgravity with PIO2 of 127 mmHg to cover the durations of Exploration Class missions, so a health monitoring and mitigation plan is required to implement this condition. These guiding PIO2 values may change as further research yields information to better define the physiological limits and acceptable duration for an alternative spaceflight system environment.]
Table 6.2-1—Inspired Oxygen Partial Pressure Exposure Ranges
6.2.1.3 Nominal Vehicle/Habitat Carbon Dioxide Levels
[V2 6004] The system shall limit the average one-hour CO2 partial pressure (ppCO2) in the habitable volume to no more than 3 mmHg.
[Rationale: Achieving this level is dependent on individual and total crew generation of CO2 for all planned activities (factoring in metabolic rates and respiratory quotient), appropriate CO2 scrubbing and adequate ventilation flow rates to ensure that no localized pockets of CO2 are generated throughout the habitat. Considerations from other payloads that generate CO2 are to be taken into account of these totals.
Note: Off-nominal CO2 values are not included within this NASA Technical Standard due to the unique circumstances of each mission (expected human performance, duration of exposure, access to medical care, etc.) and will be derived as a lower-level program/project requirement.]
6.2.2 Atmospheric Pressure
6.2.2.1 Total Pressure Tolerance Range for Indefinite Crew Exposure
[V2 6006] The system shall maintain the pressure to which the crew is exposed to between 34.5 kPa < pressure ≤ 103 kPa (5 psia < pressure ≤ 15.0 psia) for indefinite human exposure without measurable impairments to health or performance.
[Rationale: Designers and physiologists have to evaluate and trade off the various atmospheric combinations. For missions with frequent EVAs, minimizing the pressure difference between the habitat and EVA suit is preferred. Low total pressure requires a higher percentage of oxygen in the atmosphere to provide an acceptable PIO2. Oxygen-rich atmospheres, however, present safety hazards because of their ability to feed fires. The lowest pressure at which normoxia (PIO2 = 149 mmHg) is maintained at 100% O2 is 3.8 psia, however given the need to maintain a diluent gas at 30% ([V2 6002] Inert Diluent Gas) while maintaining normoxia and CO2 limits ([V2 6004] Nominal Vehicle/Habitat Carbon Dioxide Levels), the lowest achievable cabin pressure is 5.0 psia (see Figure 6.2-1—Atmospheric Composition). Total pressure must be considered in conjunction with O2 and CO2 requirements. Under certain spacesuit operations (e.g., DCS treatment, leak checks), the crewmember may be exposed to pressures above or below this range for a limited period of time.]
Figure 6.2-1—Atmospheric Composition
6.2.2.2 Rate of Pressure Change
[V2 6007] For pressure changes >1.0 psi, the rate of change of total internal vehicle pressure shall not exceed 13.5 psi/min.
[Rationale: This rate of pressure change is used across numerous environments and provides a good boundary for human tolerance. This rate of change helps decrease risk of injury to crewmembers’ ears and lungs during depressurization and repressurization but does not replace the [V2 6150] Barotrauma Prevention requirement. Microgravity may have affected head and sinus congestion and is therefore much more conservative than the 30 psi/min (1550 mmHg/min) (75 ft/min) descent rate limit allowed by the U.S. Navy Diving Manual. This rate of change limit is consistent with the U.S. Navy Diving Manual 13.5 psi/min (700 mmHg/min) (30 ft/min) ascent rate allowance, which is used primarily for DCS prevention, not barotrauma prevention. For transient pressure changes ≤ 1.0 psi, the rate of change of total internal vehicle pressure can exceed 13.5 psi/min.]
6.2.2.3 Barotrauma Prevention
[V2 6150] During a commanded pressure change, the system shall pause within 1 psi of the pause command being issued by the unsuited or suited crewmember, with ability to increase or decrease pressure as needed after the pause.
[Rationale: During all intentional pressure changes, crewmembers may need to pause the pressure change within the system allowing additional time to adjust to the change in pressure and to avoid barotrauma. If the pause does not alleviate discomfort, the crewmember needs to be able to reverse the direction of the pressure change. This could be necessary if a crewmember is unable to equalize pressure in their ears and sinuses.]
6.2.2.4 Decompression Sickness (DCS) Risk Identification
[V2 6008] Each program shall define mission unique DCS mitigation strategies to achieve the level of acceptable risk of DCS as defined below within 95% statistical confidence:
- DCS ≤ 15% (includes Type I or isolated cutis marmorata).
- Grade IV venous gas emboli (VGE) ≤ 20%.
- Prevent Type II DCS.
[Rationale: DCS risk limits have been defined to develop coordinated requirements for the habitat (e.g., vehicle, EVA suit) including total pressure, ppO2 and prebreathe before vehicle or suit depressurization, which are all variables in DCS risk. These risk limits are designed to protect crew health from severe DCS that could affect long term health: historically, <15% DCS I incidence correlates with rare DCS II (serious/neurologic/life threatening DCS) and also is associated with a high likelihood of resolution with repressurization. Specific programs/missions may require a significantly lower risk of DCS to ensure mission goals, and thus may implement a lower risk of DCS. This level of risk minimizes impact to crew long term health consequences. If DCS develops, initiating treatment as soon as possible improves the likelihood of treatment success, therefore actual or suspected DCS will most often result in EVA termination. Crewmembers also have a required 24-hr exclusion from reduced pressure activities post successful DCS treatment per JPR 1800.3E Decompression Sickness, Sections 3.1-3.3. Therefore, lower levels of risk may be necessary to minimize EVA interruption and ensure mission success. This may involve longer prebreathe times, delayed EVA mission operations or decreasing decompression stress through minimizing the change in pressure from the vehicle to the EVA suit.]
6.2.2.5 Decompression Sickness Treatment Capability
[V2 6009] The system shall provide DCS treatment capability.
[Rationale: DCS is a potential hazard of spaceflight and EVA because of changes in the operational pressure environment. Rapid and appropriate intervention is required to optimize the outcome for the affected crewmember. If treatment for DCS is instituted quickly, the outcome of therapy has a higher probability of success and will likely require less magnitude and duration of hyperbaric O2 therapy. It is important, therefore, to have the crewmember back to their initial saturation pressure as soon as possible, which may resolve DCS symptoms. Initial saturation pressure is defined as the highest pressure to which the crewmember has been exposed during the 36 hours before beginning the EVA. If not addressed quickly, higher pressures may be required to address DCS symptoms. The U.S. Navy Treatment, Table 6, Oxygen Treatment of Type II Decompression Sickness (treatment in a hyperbaric treatment facility) found in the U.S Navy Diving Manual, is the terrestrial standard for treating most forms of DCS; however, the terrestrial standard may not be achievable, or required, because the resources required to support it would be prohibitive, and the expected outcomes from sub-terrestrial standard therapy are likely to be adequate for altitude-induced DCS symptoms.]
6.2.3 Temperature and Humidity for Crew Health and Performance
6.2.3.1 Crew Health Environmental Limits
[V2 6012] The system shall maintain levels of cabin humidity and temperature within the boundaries of the Operating Limits as shown in Figure 6.2-2—Crew Health Environmental Limits, to protect for crew health during pressurized operations when crew occupies the cabin, excluding suited operations, ascent, entry, landing, and post landing.
[Rationale: The intent of the Crew Health Environmental Limits is to provide the range of cabin humidity and temperature that protects crew health. Figure 6.2-2 is based on ASHRAE 55 and anchored at 18°C and 75% humidity on the upper left corner and 27 °C and 25% humidity on the lower right corner. Operations outside of these boundaries may cause crewmembers to experience health impacts (dry, stuffy and/or irritated mucous membranes), skin rashes (due to microbial growth at high humidity levels) thermal discomfort (overheating depending on tasks), and/or decreased performance. From an engineering perspective, average humidity is to be maintained above this lower limit (25%) to ensure that the environment is not too dry to prevent static electricity buildup within the cabin, which could pose an electrical hazard to crew and a possible ignition hazard. Refer to [V2 6013] Crew Performance Environmental Zone for the range that protects for both health and performance. This requirement does not apply to EVA suits. Corresponding EVA suit requirements addressing temperature, humidity, pressure, and other elements that affect comfort in a spacesuit are defined in section 11 of this NASA Technical Standard.]
Figure 6.2-2—Crew Health Environmental Limits
6.2.3.2 Crew Performance Environmental Zone
[V2 6013] The system shall be capable of reaching atmospheric humidity and temperatures of nominally occupied habitable volumes within the zone provided in Figure 6.2-3—Crew Performance Environmental Zone, during all nominal operations, excluding suited operations, ascent, entry, landing, and post landing.
[Rationale: This temperature zone is defined as the operating zone of environmental conditions in which humans can achieve thermal comfort and not have their performance of routine activities affected by thermal stress. Operating outside of this zone will cause performance decrements and impact productivity. Due to individual variability among crewmembers and the likelihood of different crewmembers performing different tasks at different metabolic rates, it is necessary to have the capability to change temperature set points, adjust localized air flow, and/or have various clothing options available to achieve thermal comfort for all crew. Studies of office working environments and OSHA recommendations generally find these atmospheric parameters (20°C -25°C and 30-60% humidity) to support human comfort and performance (T.M. Ikaheimo, 2014; Seppanen, Fisk, & Lei, 2006; OSHA Policy on Indoor Air Quality: Office Temperature/Humidity and Environmental Tobacco Smoke, 2003).]
Figure 6.2-3—Crew Performance Environmental Zone
6.2.3.3 Crewmember Heat Storage
[V2 6014] The system shall prevent the energy stored by each crewmember from exceeding the cognitive deficit onset (CDO) limits defined by the range 4.7 kJ/kg (2.0 Btu/lb) > ΔQ stored > -4.1 kJ/kg (-1.8 Btu/lb) during pre-launch operations, ascent, entry, descent, landing, postlanding, contingency, and suited operations longer than 12 hours, where ΔQ stored is calculated using a validated and NASA-approved thermoregulatory model, such as 41-Node Man (JSC-33124, 41-Node Transient Metabolic Man Computer Program Documentation – A thermal regulatory model of the human body with environmental suit applications) or the Wissler model.
[Rationale: The intent of this requirement is to protect the crewmember from heat-related illness. Heat stored by crewmembers during launch, landing, and off-nominal suited operations may also need to be addressed as part of total system design and human accommodation. These thermal limits are intended to cover brief temperature excursions related to contingency situations, including excessively high metabolic rates, or operational exposure to excessive ambient heat loads, including suited operations.]
6.2.3.4 Temperature Selectability
[V2 6151] The system shall provide selectable set points for internal atmosphere temperature in step sizes no greater than 0.5°C increments with a setpoint error of +/- 1.5°C in the habitable volume.
[Rationale: The intent of this requirement is to allow the crew to adjust the temperature to accommodate crew performance and provide some adjustment for crew preference. It is expected that the system can be set to temperatures in the environmental operating range (per [V2 6012] Crew Health Environmental Limits) and the system will accommodate those temperatures if the capability exists.]
6.2.3.5 Temperature Adjustability
[V2 6152] The system shall be capable of adjusting temperature in the habitable volume by at least 1°C/hr.
[Rationale: The cabin temperature needs to be capable of being adjusted in a timely manner (1° Celsius per hour) in order to accommodate real-time crew activities, performance, and crew preference.]
6.2.3.6 Post Landing Relative Humidity (RH)
[V2 6011] For nominal post landing operations, the system shall limit RH to the levels in Table 6.2-2—Average Relative Humidity Exposure Limits for Post Landing Operations.
[Rationale: Average humidity is to be maintained above the lower limits stated to ensure that the environment is not too dry for the nominal functioning of mucous membranes. During low humidity exposures, additional water is to be provided to the crew to prevent dehydration. Humidity is to be maintained below the upper limits for crew comfort to allow for effective evaporation and to limit the formation of condensation. In unsuited scenarios, high RH may interfere with the nominal evaporation process that enables perspiration to cool the body. Thus, high RH in warm environments can pose an additional hazard for core body temperature excess.]
Table 6.2-2—Average Relative Humidity Exposure Limits for Post Landing Operations
6.2.4 Atmospheric Control
6.2.4.1 Atmospheric Control
[V2 6017] The system shall allow for local and remote control of atmospheric pressure, humidity, temperature, ventilation, and ppO2.
[Rationale: The ability to control atmospheric conditions is important for crew comfort, e.g., temperature and humidity, and for mission tasks, e.g., ppO2 and total pressure for expected cabin depressurization, to ensure efficient and effective performance. This requirement does not apply to spacesuits. The corresponding spacesuit requirement is defined in requirement [V2 11033] Suited Thermal Control, in this NASA Technical Standard. The ability to adjust atmospheric parameters remotely is important for cases in which a crewed vehicle is to dock with an uncrewed vehicle whose atmosphere is to be habitable before ingress. This may be done from other spacecrafts located in microgravity, extraterrestrial body surfaces, or Earth- or lunar-based control centers.]
6.2.4.2 Environmental Control During Exercise
[V2 7041] The system environmental control shall accommodate the increased O2 consumption and additional output of heat, CO2, perspiration droplets, odor, and particulates generated by the crew in an exercise area.
[Rationale: The ppO2 in the exercise area(s) is to be maintained at normal levels; otherwise, the required physiological capabilities of crewmembers may be impaired. This requirement also addresses any particulate that may be generated by the exercise activity, e.g., skin, hair, or lint from clothing or other materials.]
6.2.5 Atmospheric Data Availability
6.2.5.1 Atmospheric Data Recording
[V2 6020] For each isolatable, habitable compartment, the system shall automatically record pressure, humidity, temperature, ppO2, and ppCO2 data continuously.
[Rationale: Access to atmospheric data is needed for each habitable compartment (that can be isolated with a pressure hatch) to which the crew has access, as each of these parameters is critical to crew health and comfort. Additionally, the ability to view past recorded data helps to prevent environmental conditions that could harm the crew or vehicle and can aid in the effort to troubleshoot problems. This requirement does not apply to spacesuits. The corresponding spacesuit requirement is defined in requirement [V2 11034] Suited Atmospheric Data Recording, in this NASA Technical Standard.]
6.2.5.2 Atmospheric Data Displaying
[V2 6021] The system shall display real-time values for pressure, humidity, temperature, ppO2, and ppCO2 data to the crew locally and remotely.
[Rationale: These atmospheric parameters are critical to human health and comfort, and access to this atmospheric data needs to be provided to the crew. The crew needs to view the environmental status in real time to help prevent environmental conditions that could harm them or the vehicle. This requirement does not apply to spacesuits. The corresponding spacesuit requirement is defined in requirement [V2 11035] Suited Atmospheric Data Displaying in this NASA Technical Standard.]
6.2.6 Atmospheric Monitoring and Alerting
6.2.6.1 Atmospheric Monitoring and Alerting Parameters
[V2 6022] The system shall alert the crew locally and remotely when atmospheric parameters, including atmospheric pressure, humidity, temperature, ppO2, and ppCO2 are outside safe limits.
[Rationale: Systems are to be capable of alerting the crew when atmospheric parameters are outside set limits so the crew can take appropriate actions to maintain health and safety. See section 10.5.1 Audio Systems, in this NASA Technical Standard for additional information. This requirement does not apply to spacesuits. The corresponding spacesuit requirement is defined in requirement [V2 11036] Suited Atmospheric Monitoring and Alerting, in this NASA Technical Standard.]
6.2.6.2 Trace Constituent Monitoring and Alerting
[V2 6023] The system shall monitor trace volatile organic compounds (VOCs) in the cabin atmosphere and alert the crew locally and remotely when they are approaching defined limits.
[Rationale: Monitoring and alerting are required to identify when hazardous contaminants are detected and to alert the crew so they can take appropriate actions to maintain health and safety. Trace contaminant monitoring is important for identifying a wide range of contaminants that may impact human health and safety, including toxic substances that cannot be predicted now or substances that are not normally thought of as part of the atmosphere. Accepted limits may be based on JSC-20584, Spacecraft Maximum Allowable Concentrations (SMACs), or on agreements from international partners. There may be specific mission scenarios (e.g., shortduration missions and alternate controls) where trace contaminant monitoring may not be required.]
6.2.6.3 Combustion Monitoring and Alerting
[V2 6024] The system shall monitor in real-time the toxic atmospheric components listed in Table 6.2-3—Recommended Combustion Product (CP) Monitoring Ranges, that would result from pre-combustion and combustion events in the ranges and with the accuracy and resolution specified in the table, and alert the crew locally and remotely in sufficient time for them to take appropriate action.
[Rationale: Monitoring of toxic by-products of combustion is generally used for operational decisions and response. Because of the extreme danger of combustion in a spacecraft, combustion product monitors are to be readily accessible and provide quick, stable, and accurate readings. Monitoring and alerting are required to identify when toxic combustion products are present and to notify the crew to take appropriate actions to maintain health and safety.]
Table 6.2-3—Recommended Combustion Product (CP) Monitoring Ranges
6.2.6.4 Contamination Monitoring and Alerting
[V2 6025] The system shall monitor and display atmospheric compound levels that result from contamination events, e.g., toxic release, systems leaks, or externally originated, before, during, and after an event and alert the crew locally and remotely in sufficient time for them to take appropriate action.
[Rationale: Alerting the crew when contaminants are present is necessary for them to take appropriate action to maintain health and safety. In addition, monitoring after the event is important to verify that levels are safe for human exposure. Monitoring is required to identify when components are detected so that alerting can occur. Potential contaminants, e.g., hydrazine, monomethylhydrazine (MMH), unsymmetrical dimethylhydrazine (UDMH), nitrogen tetroxide/nitrogen dioxide and ammonia, need to be monitored after EVA.]
6.2.6.5 Celestial Dust Monitoring and Alerting
[V2 6153] The vehicle shall monitor celestial dust and alert the crew locally and remotely when they are approaching defined limits.
[Rationale: Celestial dust includes, but is not limited to, lunar, Martian and other extraterrestrial bodies. In-flight monitoring of habitable environments is required to characterize concentrations of celestial dust which enables any necessary crew action to maintain health and safety, tracking of average exposure, while also informing necessary treatment options after the mission, and providing a record of crew exposures. Lunar dust monitoring frequency and particle size fraction is dependent on mission characteristics and whether crew health concerns are based on chronic or acute exposure considerations as noted in [V2 6053] Lunar Dust Contamination. There may be other specific mission scenarios (e.g., surface launch vehicle docking to orbital vehicle) where dust monitoring may be required.]
6.2.7 Atmospheric Ventilation
6.2.7.1 Nominal Vehicle/Habitat Atmospheric Ventilation
[V2 6107] The system shall maintain a ventilation rate within the internal atmosphere that is sufficient to provide circulation that prevents CO2 and thermal pockets from forming, except during suited operations, toxic cabin events, or when the crew is not inhabiting the vehicle.
[Rationale: Crew and equipment give off heat, moisture, and CO2 that will lead to parameters outside the bounds of environmental requirements if adequate ventilation is not provided. Maintaining proper ventilation within the internal atmosphere is necessary to ensure that stagnant pockets do not form, and the temperature, humidity, and atmospheric constituents are maintained within their appropriate ranges. The two-thirds value for atmosphere velocities in the requirement has historically proven to be a reasonable balance between design constraints such as power, acoustics, and safety. The effective atmosphere velocity range of 4.57-36.58 m/min (15-120 ft/min) pertains to the time averaged velocity magnitudes in the crew occupied space using averages over time periods sufficient to achieve stability. This range is considered sufficient to provide circulation that prevents CO2 and thermal pockets from forming. Cabin ventilation is not required during suited operation since the suit will provide necessary air circulation. Fire or any toxic release into the atmosphere are examples of periods during which the mentioned ventilation rates are not in the best interest of air quality and crew health. In those cases, the ventilation system may need to be shut down to protect the safety of the crew.]
6.2.7.2 Off-Nominal Vehicle/Habitat Atmospheric Ventilation
[V2 6108] The system shall control for ppO2, ppCO2, and relative humidity during off-nominal operations, for example during temporary maintenance activities in areas not in the normal habitable volume.
[Rationale: The crew may be required to perform maintenance behind a panel in an area that is not part of the normal habitable volume, and which therefore does not have ventilation. Maintaining proper ventilation within the internal atmosphere is necessary to ensure that stagnant pockets do not form, and the temperature, humidity, and atmospheric constituents are maintained within their appropriate ranges. See [V2 6003] O2 Partial Pressure Range for Crew Exposure, [V2 6004] Nominal Vehicle/Habitat Carbon Dioxide Levels, and [V2 6012] Crew Health Environmental Limits, in this NASA Technical Standard. Examples of historical ventilation techniques include equipment such as flexible (reconfigurable) ducting, portable fans, or diverters.]
6.2.8 Atmospheric Contamination
Note: For contamination, units of measure are expressed in metric units only in the sections that follow.
6.2.8.1 Atmosphere Contamination Limit
[V2 6050] The system shall limit gaseous pollutant accumulation in the habitable atmosphere below individual chemical concentration limits specified in JSC-20584, Spacecraft Maximum Allowable Concentrations for Airborne Contaminants (SMACs).
[Rationale: Exposure limits for expected airborne spacecraft contaminants are established to protect crewmembers from illness and injury. The SMACs provide guidance for short-term (1 and 24 hours), medium-term (7 and 30 days), and long-term (180 days and 1,000 days) exposure of these constituents. The SMACs take into account several unique factors of human spaceflight missions, including the stress on human physiology, the uniform good health of astronauts, and the absence of pregnant or very young individuals. Short-term SMACs are intended for offnominal events and are not to be used for system design or evaluation of routine exposures. Considerations are to be taken for scenarios where there is a period of dormancy or uncrewed operations.]
6.2.8.2 Particulate Matter
[V2 6052] The system shall limit the habitable atmosphere particulate matter concentration for total dust to <3 mg/m3 with a crew generation rate of 1.33 mg/person-minute, and the respirable fraction of the total dust <2.5 μm (micrometer) in aerodynamic diameter to <1 mg/m3 with a crew generation rate of 0.006 mg/person-minute.
[Rationale: These values were derived by applying a factor of five to the OSHA limits for nuisance dusts, which is the best analog for the ordinary dust present in spacecraft. They do not apply to reactive dust, e.g., lithium hydroxide (LiOH) or extraterrestrial dust. The factor of five is applied to adjust from intermittent occupational exposure to continuous spaceflight exposure. The basis for the particulate matter generation rates is documented in ICES-2019-58, The Impacts of Cabin Atmosphere Quality Standards and Control Loads on Atmosphere Revitalization Process Design. The generation rate for the respirable fraction of the total dust was calculated by adding the generation rate for particles <1 μm in diameter and one-half the generation rate for particles with diameters between 1 μm and 5 μm.]
6.2.8.3 Lunar Dust Contamination
V2 6053] The system shall limit the levels of lunar dust particles less than 10 μm in size in the habitable atmosphere below a time-weighted average of 0.3 mg/m3 during intermittent daily exposure periods that may persist up to 6 months in duration.
[Rationale: This limit was based on detailed peer-reviewed studies completed by the Lunar Atmosphere Dust Toxicity Assessment Group (LADTAG) and is specific to the conditions relevant to the lunar surface, i.e., this requirement would not necessarily be applicable to other missions. The requirement assumes that the exposure period is episodic and is limited to the time before ECLSS can remove the particles from the internal atmosphere (assumed as eight hours post introduction). Although the requirement is being conservatively applied to all inhalable particles (all particles ≤10 μm), it is most applicable to dusts in the respirable range (≤2.5 μm) that can deposit more deeply into the lungs. Studies show that the particle size of lunar dust generally falls within a range of 0.02-5 µm. The ability to meet this requirement will depend upon factors such as the level of lunar dust introduction and ECLSS removal rates. The monitoring of dust is captured in [V2 6153] Celestial Dust Monitoring and Alerting.]
6.2.8.4 Microbial Air Contamination
[V2 6059] The system shall provide air in the habitable atmosphere that is microbiologically safe for human health and performance.
[Rationale: Microbiologically safe air is essential to prevent infection, allergic response, and mitigate risk to crew health and performance. Assessing microbiological safety of air relies primarily on the enumeration and identification of viable, medically significant microorganisms (bacteria and fungi) that are known to cause disease. Historically, atmospheric microbial concentrations in spacecraft habitable atmosphere have been controlled by maintaining a continuous flow of air through High Efficiency Particulate Air (HEPA) filters that remove at least 99.97% of airborne particles 0.3 μm in diameter, or larger. Medical significance of microorganisms and allowable levels of microorganisms are set based on guidance from the JSC Microbiology Laboratory. Program level requirements for atmospheric quality include considerations for factors such as vehicle architecture and mission duration. Considerations are to be taken for scenarios where there is a period of dormancy or uncrewed operations.]
6.3 Water
The challenges of providing quality water vary for different system designs and diverse water sources, e.g., recycled humidity condensate and ground-supplied water. In the design process, early identification of potential water quality impacts enables mitigation by design. Water quality monitoring through the use of in-flight and preflight analysis techniques are essential tools for use in verifying water quality, evaluating trends, and documenting potential exposures.
6.3.1 Water Quality and Monitoring
6.3.1.1 Potable Water Quality
[V2 6026] At the point of crew consumption or contact, the system shall provide aesthetically acceptable potable water that is chemically and microbiologically safe for human use, including drinking, food rehydration, personal hygiene, and medical needs.
[Rationale: Point of crew consumption or contact refers to the location from which potable water is dispensed for use in drinks, food rehydration, personal hygiene, medical needs, and any potential in-flight maintenance sites. Safe water pollutant levels have been established for certain prioritized compounds specifically for human-rated space vehicles by the JSC Toxicology and Environmental Chemistry Laboratory in cooperation with a subcommittee of the National Research Council Committee on Toxicology; however, the current list in JSC-63414, Spacecraft Water Exposure Guidelines (SWEGs), is not all inclusive, and other compounds may be of concern. For these other compounds, the United States Environmental Protection Agency maximum contaminant levels can be utilized as conservative screening limits. For additional guidance, reference chapter 6, Natural and Induced Environments, in the Human Integration Design Handbook (HIDH). To determine which contaminants are present, a complete chemical characterization of potential water sources is to be performed. Evaluation of aesthetic properties is important to ensure that the potable water does not have an adverse odor or taste such that it would cause crewmembers to diminish consumption and increase the risk of underhydration or dehydration of the crewmember. Aesthetic acceptability can either be assessed qualitatively by an evaluation panel or indirectly through compliance with the applicable water quality requirements.
Special considerations need to be taken with treatment chemicals and residual biocides. For example, at effective biocidal levels iodine can alter the aesthetics of the water as well as cause iodine-related illness; refer to Section 6.3 of the HIDH, NASA/SP-2010-3407. Allowable concentrations for other treatment chemicals and biocides can be found in JSC-63414 and United States Environmental Protection Agency guidelines.
Microbially safe water is essential to prevent infection and mitigate risk to crew health and performance. Microbiological assessment of water safety relies primarily on the identification of viable, medically significant microorganisms (bacteria, fungi, and parasitic protozoa) that are known to cause disease. Monitoring targets have included specific categories of microorganisms (e.g., coliform bacteria) that indicate that the water may have been contaminated or not adequately treated. Microbial enumeration of potable water has also been used historically as an indicator of overall system performance. Previous spaceflight missions have used these monitoring approaches in combination with mitigation strategies to confirm the safety of potable water. Medical significance of microorganisms and allowable levels of microorganisms are set based on guidance from the JSC Microbiology Laboratory. Program level requirements for water quality include considerations for factors such as water source, vehicle architecture, and mission duration.]
6.3.1.2 Water Contamination Control
[V2 6051] The system shall prevent potable and hygiene water supply contamination from microbial, atmospheric (including dust), chemical, and non-potable water sources to ensure that potable and hygiene water are provided.
[Rationale: While ensuring the delivery of potable water to crew on orbit is important, contamination from sources within the delivery system or from the environment is also possible.]
6.3.1.3 Water Quality Monitoring and Alerting
[V2 6046] The system shall provide the capability to monitor water quality and notify the crew locally and remotely when parameters are approaching defined limits.
[Rationale: On-orbit water quality is critical to the health of the crew as required in [V2 6026] Potable Water Quality. Rigorous ground processing with preflight water sampling and contamination assessment prevents in-flight water quality problems and thus minimizes the need for in-flight contamination monitoring and remediation of any water quality parameters that are out of specification. In-flight sampling capability supports real-time contaminant monitoring and remediation of stored or regenerated water systems as needed for long-duration missions. Ground-based quality analyses of in-flight and post landing samples provide a record of crew exposures and are used to determine follow-on ground processing steps.]
6.3.2 Water Quantity
[V2 6109] The system shall provide a minimum water quantity as specified in Table 6.3-1— Water Quantities and Temperatures, for the expected needs of each mission, which are considered mutually independent.
[Rationale: To maintain crewmember hydration status and allow crewmembers to perform duties nominally, adequate water intake is needed, which is a culmination of drinking water, dietary intake from food and drinks, as well as fluid loading and recovery needs. Proper hydration contributes to adequate urine output to clear metabolic wastes and to account for perspiratory and other insensible losses. Dehydration of the crewmember will have consequences ranging from poor communication and crewmember performance caused by dry mucous membranes, nosebleeds, headache, malaise, and fitful sleep to urinary tract infection or urinary calculi if the under-hydration state is continued. Dehydration also cancels many of the thermal benefits of heat acclimatization and aerobic fitness. Potable water is also necessary during suited operations to prevent dehydration related to perspiration and insensible water loss, as well as to improve comfort.
For fluid loading, 1.5 L of water per crewmember is based on the Space Shuttle and the Commercial Crew Program (CCP) prescriptions for reentry fluid loading. Table 6.3-1 calls out a minimum of 1 L (33.8 fl oz) of water per crewmember, and an additional 500 mL (16.9 fl oz) will be available per crewmember from their unused daily water allocation. Vehicles that may wave off prior to reentry need to protect for two fluid loadings per crewmember. Fluid loading is based on crewmember weight and requires the use of salt tablets in addition to water unless an alternative solution is used. Without fluid loading, the crew is more likely to experience orthostatic intolerance (passing out when standing) during and after deorbit.
Of note, the water required during the post landing phase, for up to about 36 hours, is to ensure crewmembers are properly hydrated. Less water may be needed for hydration following a launch abort, since crewmembers will not have undergone spaceflight fluid loss. Additionally, for missions longer than a few days, hot and cold food and beverages provide an important psychological benefit. The amount of hot and cold water to be provided depends on the number of crew, mission length, and types of food and beverage available. To ensure the crew have adequate hydration, the Potable Water for Hydration minimum amount per day is available for drinking, and for rehydrating foods/drinks. This value does not change with removal of rehydratable foods or addition of thermostabilized food as the minimum amount is based on the physiological needs and not how it is consumed.
Water is also needed for personal hygiene, which will depend on the mission length, number of crewmembers, and design of the hygiene system. Clean water is necessary for maintaining skin, hair, and dental health of the crew. Water may not be required for some hygiene activities where alternatives, e.g., rinseless shampoo, pre-wetted towels, are provided. Water for medical contingency use is required for many situations, including wound irrigation during the various activities of a mission, which is based on experience and data from Shuttle, ISS, and Apollo programs. The amount of contingency water will be determined on the expected events to occur and frequency of events. Eye wash capability for particulate events is expected, especially for lunar and planetary missions, as there is an increased risk of exposure to dust and regolith on the lunar or other planetary surfaces. Some medical situations require large quantities of water, for example, LiOH or other toxic substances in the eye or skin, or in a wound. However, these events are off-nominal and occur at lower frequency during the mission than particulate events and may be considered contingencies. The quantity of water to be provided depends on the number of crewmembers, duration of mission, and expected contingency events and should ensure that medical treatment can be provided.
Additional considerations for water quantities include sampling needs for routine water testing and monitoring, as well as any agricultural or hydroponic systems that would be in addition to the crew needs for hydration. All quantities are considered mutually independent. Water quantities provided are not limited to only what is replenished with the arrival of cargo or crew vehicles and can be obtained by other means, including that of recycling.]
6.3.3 Water Temperature
[V2 6110] The system shall provide the appropriate water temperature as specified in Table 6.31—Water Quantities and Temperatures, for the expected needs of each mission and task.
[Rationale: Over the course of long-duration missions, crewmembers can tire of repetitive beverages and foods. Providing hot and cold water is an important way of keeping the crewmembers interested in their meals and providing a familiar contact to normal Earth living, as well as making the food items more acceptable and palatable. During the Apollo missions, it was noted that the crew reported that there was a need for hot water while in the lander to not only provide warmth, but as comfort during the missions. To aid in the well-being and for any food/drinks consumed, a portion (600 mL) of the potable water will need to be available as hot water for immediate use. However, the minimum water needed per crewmember per day is 2.5 L (84.5 fl oz), regardless of the portions allocated as hot, cold or room temperature. Additionally, the use of higher water temperatures allows for faster rehydration of beverages and foods, as well as aiding in the prevention of microbial growth.
Providing proper temperature of hygiene and medically used waters will support comfortable body cleansing and prevent thermal injury to the tissue, especially when performing irrigations. Terrestrially, building code for typical shower valves is to limit the outlet temperature to 120°F (48.9C), which is at the tissue damage limit. Note that the average ISS Potable Water Dispenser (PWD) hot water is 180°F (~82°C) but can vary from 150-200°F (~65-93°C). Crewmembers are trained to use a combination of Ambient and Hot to get the desired temperature for hygiene.]
Table 6.3-1—Water Quantities and Temperatures
6.3.4 Water Dispensing
6.3.4.1 Potable Water Dispensing Rate
[V2 6039] Water shall be dispensed at a rate that is compatible with the food system.
[Rationale: A water dispensing rate is to be defined as a rate that is compatible with the food packaging and time demands of the allotted meal schedule to ensure that the crew is able to prepare for and perform tasks, e.g., filling drink bags and/or rehydrating food in a reasonable amount of time. The rate will depend on the design of the food system and the amount of water required, if necessary, to rehydrate beverages and food. The program will define appropriate increments in accordance with HMTA. This rate is not intended to require an additional water quantity beyond that required for nominal mission water usage. It needs to be compatible with the increments, for example a rate of 500 mL/minute (16.9 oz/minute) can ensure timely food preparation when rehydration is needed for multiple meals or drinks for all crewmembers and meals.]
6.3.4.2 Potable Water Dispensing Increments
[V2 6040] To prevent overflow, water shall be dispensable in specified increments that are compatible with the food preparation instructions and time demands of the allotted meal schedule.
[Rationale: Water dispensing increments are to be defined to properly hydrate food and beverages. In addition, palatability is to be included as part of the assessment when determining the proper hydration of food and beverages. On ISS today, water is dispensed at 25 mL (milliliter) increments. All food portions are sized to be compatible with this increment (i.e., 25 mL to 250 mL). The increment was different in previous missions, as low as 15 mL. The program will define appropriate increments in accordance with HMTA.]
6.4 Environmental Hazards
The system interior atmosphere, water, or surfaces can become contaminated from multiple inflight sources during operations, including material offgassing, payloads, other vehicles, crew, and planetary environments. Accordingly, only those materials or substances that, if offgassed or released into the habitable volume, do not or will not form hazardous substances and would not threaten human health, are to be used within the spacecraft.
6.4.1 Toxic Chemicals
6.4.1.1 Toxic Hazard Level Three
[V2 6047] The system shall use only chemicals that are Toxic Hazard Level Three or below, as defined in JSC-26895, Guidelines for Assessing the Toxic Hazard of Spacecraft Chemicals and Test Materials, in the habitable volume of the spacecraft.
[Rationale: Potential contamination assessed as Toxic Hazard Level Four cannot be cleaned up by the crew and pose a risk of permanent injury or death. As such, only materials that either pose limited risk to crew or can be contained and disposed of by an appropriate clean-up procedure should be used in the habitable volume or in systems that may credibly be released into the habitable volume (i.e., thermal working fluids). Toxic Hazard Level ratings are assigned by JSC Toxicology based on information received per JSC-27472, Requirements for Submission of Data Needed for Toxicological Assessment of Chemicals to be Flown on Manned Spacecraft.]
6.4.1.2 Toxic Hazard Level Four
[V2 6048] The system shall prevent Toxic Hazard Level Four chemicals, as defined in JSC26895, Guidelines for Assessing the Toxic Hazard of Spacecraft Chemicals and Test Materials, from entering the habitable volume of the spacecraft.
[Rationale: Potential contamination assessed as Toxic Hazard Level Four cannot be contained by crew and may cause appreciable effects on coordination, perception, memory, long-term serious injury (e.g., cancer), or may result in internal tissue damage. Toxic Hazard Level ratings are assigned by JSC Toxicology based on information received per JSC-27472, Requirements for Submission of Data Needed for Toxicological Assessment of Chemicals to be Flown on Crewed Spacecraft. Utilization of THL-4 chemicals may be acceptable in the external environment provided they are not brought inside habitable environment, do not contaminate and/or damage hardware that is brought inside, and/or damage other external end items.]
6.4.1.3 Chemical Decomposition
[V2 6049] The system shall use only chemicals that, if released into the habitable volume, do not decompose into hazardous compounds that would threaten health during any phase of operations.
[Rationale: Only a few compounds, such as fluorinated coolants, have been shown to decompose into hazardous compounds during nominal spacecraft Atmosphere Revitalization System (ARS) operation. These compounds could present a toxic threat if the amount of the compound involved is sufficient, the ARS temperature is adequate, and the product compound is hazardous. Halon is an example of such a chemical; if it is sufficiently heated during its normal use as a fire suppressant, it breaks down into highly toxic gaseous compounds.]
6.4.2 Cross-Contamination Prevention
6.4.2.1 Biological Payloads
[V2 6060] Biological payloads, as well as the associated operational procedures and supporting personal protective equipment, shall meet the criteria defined by the JSC Biosafety Review Board guidelines contained in JPR-1800.5, Biosafety Review Board Operations and Requirements.
[Rationale: Biohazardous agents, which include bacteria, fungi, protozoa, viruses, cell cultures, and recombinant deoxyribonucleic acid (DNA), may be infectious and result in disease or contamination of water and food supplies or the internal environment. Payloads that contain biohazardous materials are to ensure that these materials are properly contained, handled, and discarded. JPR-1800.5 establishes requirements for the identification and assessment of biohazardous materials used in payload or ground-based experiments.]
6.4.2.2 Environment Cross-Contamination
[V2 6061] The system shall provide controls to prevent or minimize (as appropriate) biological cross-contamination between crew, payloads and vehicles to acceptable levels in accordance with the biosafety levels (BSL) defined in JPR-1800.5, as well between crew, payloads, vehicles and extraterrestrial planetary environments with the extent of application specific to individual planetary bodies and special locations thereon.
[Rationale: Biological contamination from crew, payloads (e.g., animals, plants, microorganisms), vehicle systems, waste, suits, spacecraft vehicle, and planetary environments can negatively affect crew health, performance of the vehicle and its systems, as well as the collection and integrity of scientific data (including the potential detection of microbial life forms). Additionally, biological contamination may risk planetary protection of the Earth via back-contamination upon return. Vehicle design and capability considerations related to the disposition of the crewmember remains as stated in [V1 3053] Crew Mortality Remains Disposition and [V1 3054] In Situ Disposition of Deceased Crewmember Remains in Volume 1 of this NASA Technical Standard will need to be included. Planetary protection categories and guidance to determine the applicability and extent of planetary protection measures for extraterrestrial environments as well as for return to Earth are documented in NPD 8020.7, Biological Contamination Control for Outbound and Inbound Planetary Spacecraft, and NID 8715.129 (or superseding documents) for the Moon and Mars. These documents are founded on, and consistent with the Committee for Space Research (COSPAR) Policy for Planetary Protection, an accepted approach for complying with the Treaty on Principles Governing the Activities of States in the Exploration and Use of Outer Space, including the Moon and Other Celestial Bodies (UN, 1967), Article IX.]
6.4.3 Availability of Environmental Hazards Information
[V2 6062] The system shall provide toxicological and environmental hazard information in formats accessible by the crew and ground personnel throughout the mission.
[Rationale: In case of accidental contact with hazardous materials during a mission, crew and ground personnel having access to hazard information, e.g., Safety Data Sheets (SDSs), is necessary to determine methods of cleanup and exposure treatment.]
6.4.4 Contamination Cleanup
[V2 6063] The system shall provide a means to remove or isolate released chemical and biological contaminants and to return the environment to a safe condition.
[Rationale: In the event of a contamination event, contaminants are to be removed, isolated, or reduced from the environment to ensure the crew’s health and ability to continue the mission. In some cases, such as a spill or leak, vehicle systems may be unable to remove the contaminant; and the crewmembers will have to perform the cleanup themselves. Cleanup of a contamination includes the control and disposition of the contamination.]
6.5 Acceleration and Dynamic Loads
Exceeding acceleration limits can significantly impair human performance and cause injury, thereby threatening mission success and crew survival.
For mission durations > 30 days, the crew is considered deconditioned. For mission durations ≤ 30 days, the crew is considered non-deconditioned. Emergency conditions refer to off-nominal conditions within first 30 days of Earth launch where crew survival is prioritized (e.g., launch aborts, emergency entry). The following coordinate frame as seen in Figure 6.5-1—Common XYZ Coordinate Frame for Translational Acceleration, oriented with respect to the crewmember, is employed for these requirements:
- X-Direction (Longitudinal, Forward/Aft)
- +X: Body accelerations forward/Eyes back
- -X: Body accelerations backwards/Eyes forward
- Y-Direction (Lateral, Side to Side)
- +Y: Body accelerations left/Eyes right
- -Y: Body accelerations right/Eyes left
- Z-Direction (Vertical, Up/Down)
- +Z: Body accelerations up/Eyes down
- -Z: Body accelerations down/Eyes up
Figure 6.5-1—Common XYZ Coordinate Frame for Translational Acceleration
6.5.1 Sustained Translational Acceleration Limits
[V2 6064] The system shall limit the magnitude, direction, and duration of crew exposure to sustained (> 0.5 seconds) translational acceleration by staying below the limits in Figures 6.5-(27) and Tables 6.5-(1-6) for seated and standing postures.
[Rationale: The limits in these figures represent safe levels of sustained translational acceleration under nominal and off-nominal conditions. Exposure to acceleration above these limits could significantly affect human performance for maneuvering and interacting with a spacecraft. A separate limit is defined for deconditioned crew because crewmembers are expected to have degraded capabilities due to deconditioning from exposure to reduced gravity. For the extreme conditions of a launch abort or emergency entry, limits are higher because it may be necessary to expose the crew to accelerations more severe than those experienced nominally. Humans are never to be exposed to translational acceleration rates greater than these elevated limits, as this significantly increases the risk of incapacitation, thereby threatening crew survival. The acceleration vectors are relative to the “axis” of the upper body, particularly with a focus on a line running from the eye to the heart with the neck in a neutral posture. However, the acceleration limit charts do not account for all body types or temporary off-axis accelerations or body positions. Each axis is to be analyzed separately, and conservatism in the limits for each axis covers any cumulative effect of acceleration in multiple axes.
All limits further assume adequate restraint(s) are provided for all body postures during the period of sustained loading. Adequate restraint for the purposes of Linear Sustained Acceleration Limits are defined as devices sufficient to arrest motion between the occupant and vehicle interior by applying counterforce. Restraints must also prevent unintended contact between the crewmember and the interior of the vehicle within the linear sustained acceleration limits described herein, while facilitating continual access to and operation of vehicle displays and controls. All limits also assume the crew is wearing a counterpressure garment intended to mitigate the effects of orthostatic intolerance.
For applying the standing limits refer to NASA/TM-20205008196 Artemis Sustained
Translational Acceleration Limits: Human Tolerance Evidence from Apollo to International Space Station.]
Table 6.5-1—Ax Sustained Translational Acceleration Limits (Seated)
Figure 6.5-2—Ax Sustained Translational Acceleration Limits (Seated)
Table 6.5-2—Ay Sustained Translational Acceleration Limits (Seated)
Figure 6.5-3—Ay Sustained Translational Acceleration Limits (Seated)
Table 6.5-3—Az Sustained Translational Acceleration Limits (Seated)
Figure 6.5-4—Az Sustained Translational Acceleration Limits (Seated)
Table 6.5-4—Ax Sustained Translational Acceleration Limits (Standing)
Figure 6.5-5—Ax Sustained Translational Acceleration Limits (Standing)
Table 6.5-5—Ay Sustained Translational Acceleration Limits (Standing)
Acceleration limits for deconditioned crew (standing)
Figure 6.5-6—Ay Sustained Translational Acceleration Limits (Standing)
Table 6.5-6—Az Sustained Translational Acceleration Limits (Standing)
Figure 6.5-7—Az Sustained Translational Acceleration Limits (Standing)
6.5.2 Rotation Limits
6.5.2.1 Rotational Velocity
[V2 6065] The system shall limit crew exposure to rotational velocities in yaw, pitch, and roll by staying below the limits specified in Figure 6.5-8—Rotational Velocity Limits and Table 6.5-7— Rotational Velocity Limits.
[Rationale: The limits in this figure represent safe levels of sustained rotational acceleration for crewmembers under nominal and off-nominal conditions. Exposure to rotational acceleration above these limits could significantly affect human performance for maneuvering and interacting with a spacecraft. The limits for deconditioned crewmembers are lower because crewmembers are expected to have degraded capabilities due to deconditioning from exposure to reduced gravity. For emergency conditions, limits are higher because it may be necessary to expose the crew to accelerations more severe than those experienced nominally. Humans are never to be exposed to rotational acceleration rates greater than these elevated limits as this significantly increases the risk of incapacitation, thereby threatening crew survival.]
Table 6.5-7—Rotational Velocity Limits Data for Curves
Figure 6.5-8—Rotational Velocity Limits
6.5.2.2 Sustained Rotational Acceleration Due to Cross-Coupled Rotation
[V2 6066] The system shall prevent the crew exposure to sustained (> 0.5 second) rotational accelerations caused by cross-coupled rotations greater than 2 rad/s2.
[Rationale: Crewmembers are not expected to be able to tolerate sustained cross-coupled rotational accelerations (simultaneous rotations about two different axes) in excess of 2 rad/s2 without significant discomfort and disorientation. Sustained cross-coupled rotational accelerations exceeding this amount have been found to significantly impact human performance and autonomic function (e.g., nausea, dizziness, and disorientation; degradations in neurovestibular and sensorimotor performance, physical reach, and cognition), potentially for an extended period of time after exposure. Note that rotational acceleration due to cross-coupled rotation can be computed for rotational velocity components represented in any vehicle- or head-referenced coordinate frame. Ideally, rotational velocities are decomposed into their orthogonal principal components before computing the acceleration due to cross-product terms. For scientific references regarding this subject, see chapter 6, Natural and Induced Environments, of the Human Integration Design Handbook (HIDH).]
6.5.2.3 Transient Rotational Acceleration
[V2 6067] The system shall limit transient (≤0.5 seconds) rotational accelerations in yaw, pitch, or roll as specified in Table 6.5-8—Head CG Rotational Acceleration Limits, to which the crew is exposed. The limits are appropriately scaled for each crewmember size from the 50th percentile male limits of 2,200 rad/s2 for nominal and 3,800 rad/s2 for off-nominal cases.
[Rationale: Crewmembers are not expected to be able to tolerate sustained rotational accelerations in excess of 2,200 rad/s2 for nominal and 3,800 rad/s2 for off nominal cases for a 50th percentile male. This could occur as a result of an impact, whereby brief, high-magnitude rotational accelerations are imparted to the crew. These values relate to a risk of 5% or 19% risk of brain injury, respectively, for a 50th percentile male. These values are to be appropriately scaled to other crewmember sizes as needed. For additional information scaling these limits, see Petitjean, A., et al. (2015). Normalization and Scaling for Human Response Corridors and Development of Injury Risk Curves. Accidental Injury: Biomechanics and Prevention. N. Yoganadan, A. Nahum and J. Melvin. New York, Springer Science+Business Media: 769-792.]
Table 6.5-8—Head CG Rotational Acceleration Limits
6.5.3 Acceleration Injury Prevention
[V2 6069] The system shall mitigate the risk of injury to crewmembers caused by accelerations during dynamic mission phases per Table 6.5-9—Acceptable Injury Risk Due to Dynamic Loads.
[Rationale: During dynamic flight phases, there is potential for impact and flail injury, which includes crewmember extremities impacting vehicular surfaces or objects, hyperextending, hyper-flexing, hyper-rotating, fracturing, or dislocating if proper restraints and supports are not used. Features such as harnesses, form-fitting seats, and tethers may help maintain the proper position of the crewmember’s body and limbs to reduce movement or contact with vehicle surfaces that would produce injury. In addition, the design of spacesuits may contribute to reducing injury to the crew. Preventing the inadvertent contact of extremities with vehicular structure or interior components significantly reduces the likelihood of limb fracture or soft tissue injury during a dynamic flight event. Extremity guards, tethers, garters, and handholds have been used to reduce injury in other spacecraft, aircraft, and automotive vehicles. Injury classifications are based on the Operationally Relevant Injury Scale defined in NASA/TM-2014217383, Defining NASA Risk Guidelines for Capsule-based Spacecraft Occupant Injuries Resulting from Launch, Abort, and Landing. Occurrence of dynamic cases identified in Table 6.5-9-—Acceptable Injury Risk Due to Dynamic Loads are based on statistical modeling of possible dynamic events. See NASA/TM-2014-217383 for more information. For example, during the reentry of Space Transportation System (STS)-107, the crew had notable flail injuries in their extremities, upper body, and head from the dynamic accelerations and motions that were determined to be a lethal event. Contributing factors to this involved both inertial reels from the seat belts and the helmet when the crew were exposed to cyclical motions. The seat belt inertial reel mechanisms failed, which resulted in crew body flail. The helmets did not conform to crew head, which resulted in lethal head injuries when exposed to cyclical forces.]
Table 6.5-9—Acceptable Injury Risk Due to Dynamic Loads
6.5.4 Injury Risk Criterion
[V2 6070] The system shall limit crew exposure to transient translational acceleration (≤0.5 seconds) by limiting the injury risk criterion (β/beta) to no greater than 1.0 (Low) for seated or standing crew as defined by Dynamic Response (DR) limits in NASA/TM-20205008198 Table 2 “Updated Dynamic Response Limits for Standing”, while crew are restrained as required in NASA/TM-2013-217380REV1 for seated crew, or NASA/TM – 20205008198 for standing crew.
[Rationale: The Brinkley Dynamic Response model will provide an injury risk assessment during dynamic phases of flight for accelerations <0.5 second. Application of this model assumes that a crewmember will be similarly restrained during all events where the Brinkley model is applied. Human tolerance for injury risk limits for development of space vehicles that are based on human volunteer impact test data and operational emergency escape system experience such as the Brinkley criterion have been adjusted for landing impact after reentry considering existing knowledge of physiological deconditioning related to long-term exposure to the microgravity of space. The vast experience in human testing of aircraft ejection seats and operational experience with emergency escape systems have enabled the highest fidelity for injury prediction, using the Brinkley model in the Az axis. Although the maximum allowable Brinkley β value is 1.0 for any given level of risk, the vehicle occupant protection system design is to strive to achieve β values as low as reasonably achievable for as many of the landing conditions and scenarios as possible. The criteria include dynamic response limits that have been established for varying probabilities of injury. This model may be used primarily for landing scenarios, but it is applicable for all dynamic phases of flight for accelerations less than 0.5 second. Application of the Brinkley Dynamic Response model is described in NASA/TM2013-217380REV1. Structural failure may present an occupant protection hazard through impinging upon occupant volume in such a way as to injure crewmembers.
The threshold for what constitutes standing posture is less than 80% of the total +Az axis force being transmitted through the crewmember’s buttock and thighs. Crewmembers in a standing posture without the protections offered by a seat or similar support structure will have lower tolerance to transient acceleration. Lower acceleration limits have been established to account for the risk of injury to the lower extremities. For transient accelerations occurring more than 30 days mission elapsed time, a lower limit is specified to account for spaceflight deconditioning effects on injury tolerance. These additional limits assume additional equipment mass, such as the spacesuit, borne by the crewmember is less than 20% of the crewmember’s shirtsleeve mass. They also assume sufficient extremity and torso restraints to prevent flail and motion that could result in further injury not accounted for by the reduced limits. It is assumed that the primary direction of loading will be in +Az direction for the standing posture, and that the crewmember will remain in an upright orientation during the dynamic event, as it assumed the load would be primarily in the +Az direction. The limits specified in NASA/TM-20205008198, Table 2 “Updated Dynamic Response Limits for Standing” are only valid if the restraint configuration prevents the crewmember from losing balance during the dynamic event.]
6.5.5 Dynamic Mission Phases Monitoring and Analysis
[V2 6111] The system shall collect vehicle and crewmember acceleration parameters, specific kinematic responses, and associated metadata, during all dynamic mission phases and suited operations (defined as ascent, abort, entry, descent, landing, postlanding, and EVA operations) to correlate with any injuries incurred by crewmembers.
[Rationale: Systems are to be capable of monitoring the vehicle and crewmember-specific acceleration levels and associated crewmember kinematic responses (e.g., in-cabin video, crewmember-worn accelerometry, suit instrumentation) during dynamic mission phases to correlate with any crewmember injuries. This assessment is critical to understanding the loads imparted to the human to: 1) aid in the assessment of any injuries incurred; 2) predict and correct harmful conditions before they occur; and 3) identify appropriate design changes to minimize injuries on future flights/vehicles. This data is also critical to inform modeling and analysis capabilities for future vehicles. The collection of associated metadata will enable mitigation of risks associated with dynamic mission phases, including the ability of the crew to egress during off-nominal events. Such metadata may include, but is not limited to: Vehiclerelated data such as vehicle position at landing (upright/side-lying/inverted), cabin temperature and humidity, duration of crew egress from the vehicle, and any anomalies that occurred during the dynamic phase (e.g., toxin leaks, hardware/software failures, etc.); environmental data such as wave height for water landings, time of day, and weather conditions; and physiological data such as percentage of crew with nausea/vomiting, crew fatigue and fitness level, crew postlanding task performance data, and any injuries or close-calls. Accelerometers and other sensors can be located in the suit, in the seat, or elsewhere in the vehicle.]
6.5.6 Hang Time Limit
[V2 6112] The system shall limit crew exposure to suspension trauma conditions to seven minutes or less.
[Rationale: The hang time tolerance limit of seven minutes was chosen to protect the crew from a variety of life-threatening post landing complications when their vehicle lands in an inverted posture. This time limit reduces the probability the crew will experience suspension trauma symptoms. Suspension trauma, or harness hang syndrome, is the closest comparable terrestrial condition to the crew hanging in an inverted seated position. Spaceflight medicine literature review identified that cognitive deficits could occur within three and a half minutes for a deconditioned crew, affecting their ability to take action if required. They also concluded that uprighting must be complete within seven minutes to prevent a decrease in blood pressure and loss of consciousness to the crew. This could be met through a variety of mechanisms in addition to uprighting, such as allowing crewmember seat egress. See Barr, Y & Fogarty, J (2010). Assessment of Prone Positioning of Restrained, Seated Crewmembers in a Post Landing Stable 2 Orion Configuration, JSC-CN-19414.]
6.5.7 Crew Limits in Launch Orientation
[V2 6113] The time in which crewmembers are on back with feet elevated in a launch configuration shall not exceed 3 hours and 15 minutes, excluding subsequent safing and egress time.
[Rationale: This position can be extremely fatiguing, painful, and cause musculoskeletal discomfort and difficulty urinating. These effects have the potential to impair a crewmember’s ability to perform launch duties, emergency egress procedures, and post launch tasks, and, coupled with the length of the crew day, can also affect cognitive performance. The time interval ends with lift-off. The “crew time on back” is the period from the first crewmember’s adoption of an on-back posture until launch or, in the event of a scrub, until vehicle safing begins. This time accounts for crew ingress and hold time leading to the planned launch time. It does not include vehicle safing or egress time.]
6.5.8 Extraterrestrial Surface Transport Vehicles
Crewmembers will experience accelerations and dynamic loads while operating or traversing in an extraterrestrial surface transport vehicle (ESTV). Considerations must be taken to ensure crew health and safety in these circumstances.
6.5.8.1 Extraterrestrial Surface Transport Vehicle Sustained Translation Acceleration
Limits
[V2 6154] The extraterrestrial surface transport vehicle (ESTV) system shall limit the magnitude of crewmember exposure to sustained (>0.5 seconds) translational acceleration by staying below the limits in Table 6.5-10—Extraterrestrial Surface Transport Vehicle Sustained Translation Acceleration Limits, which specify the ±Ax, ±Ay, and ±Az translational acceleration limits appropriate for specific restraint conditions.
[Rationale: When addressing the sustained translational acceleration limits, several considerations and general assumptions have been made. Further information can be found in NASA/TM-20220011974 Lunar Transport Vehicle Occupant Protection Requirements. These limits in Table 6.5-10—Extraterrestrial Surface Transport Vehicle Sustained Translation Acceleration Limits assume no intra-suit restraints other than a standard harness as seen in previous iterations of the EMU-style suit configurations. Additional occupant restraints in the form of inserts and suit modifications were not considered. With the existing suit designs at NASA, there are insufficient head and neck restraints to qualify for NASA Category 4 (K0000370695-GEN) acceleration limits.
In addition to crew health limits, the accelerations must permit the occupant to meet performance requirements. The occupant must be able to interface with the ESTV to operate the vehicle and read any instrumentation while traversing the extraterrestrial surface. It is assumed that the vehicle shall provide proper foot restraints and back support in the proposed design and shall have an occupant envelope to restrict the crew from contacting each other laterally.
The limits in Table 6.5-10 represent safe levels of sustained translational acceleration under nominal and off-nominal conditions. Exposure to accelerations above these limits could create a risk of occupant injury and significantly affect human performance for maneuvering and interacting with the human transport vehicle. The physics of the environment will limit the exposure duration to Az making the specified limits acceptable. The Ax and Ay limits must be met by a combination of vehicle design and conops (planned time versus distance of vehicle transits taking into consideration the traversed extraterrestrial terrain) which will limit exposure magnitude and duration.
These limits cover all accelerations imposed on the crewmember both directly by the human transport vehicle as well as indirectly by the ESTV through the suit.
Design solutions are expected to contain energy attenuation to mitigate the accelerations the crewmember is subjected to.
Any induced linear acceleration from rotation is subject to this requirement and must be verified.]
Table 6.5-10—Extraterrestrial Surface Transport Vehicle Sustained Translation Acceleration Limits
6.5.8.2 Extraterrestrial Surface Transport Vehicle Translation Jerk Limits
[V2 6155] The extraterrestrial surface transport vehicle (ESTV) system shall limit the crewmember exposure to translational jerk to the limits given in Table 6.5-11—Extraterrestrial Surface Transport Vehicle Translation Jerk Limits, which specifies the ±Ax, ±Ay, and ±Az translational jerk limits appropriate for specific restraint conditions.
[Rationale: When addressing the sustained translational jerk limits, several considerations and general assumptions have been made. Further information can be found in NASA/TM-
20220011974 Lunar Transport Vehicle Occupant Protection Requirements. These limits in Table 6.5-11—Extraterrestrial Surface Transport Vehicle Translation Jerk Limits assume no intra-suit restraints other than a standard harness as seen in previous iterations of the EMU-style suit configurations. Additional occupant restraints in the form of inserts and suit modifications were not considered. With the existing suit designs at NASA, there are insufficient head and neck restraints to qualify for NASA Category 4 (K0000370695-GEN) jerk limits.
Following ASTM F2291-18 guidance, the jerk limits were obtained by taking the differences between the +/- acceleration limits for the corresponding NASA Category in the acceleration table and dividing the difference by the respective body axis’s minimum onset duration (200ms for x and y axes, 133ms for z axis).
In addition to crew health limits, the experienced jerk must permit the occupant to meet performance requirements. The occupant must be able to interface with the ESTV to operate the vehicle and read any instrumentation while traversing the surface.
It is assumed that the vehicle shall provide proper foot restraints and back support in the proposed design and shall have an occupant envelope to restrict the crew from contacting each other laterally.
The limits in Table 6.5-11 represent safe levels of translational jerk under nominal and offnominal conditions. Exposure to jerk above these limits could create a risk of occupant injury in the human transport vehicle. The limits must be met by a combination of vehicle design (e.g., shock absorbers) and conops (planned time versus distance of vehicle transits taking into consideration the traversed extraterrestrial terrain).
These limits cover all jerk imposed on the crewmember both directly by the ESTV as well as indirectly by the ESTV through the suit.
Design solutions are expected to contain energy attenuation to mitigate the jerk the crewmember is subjected to.]
Table 6.5-11—Extraterrestrial Surface Transport Vehicle Translation Jerk Limits
6.5.8.3 Blunt Trauma Limits for Enabling Performance
[V2 6156] The system shall limit the crewmember exposure to blunt trauma forces to the limits given in Figure 6.5-9—Performance Blunt Force Maximum Allowable Depth of Compression Limits (Seated or Standing Vehicle Occupants), which specifies the maximum allowable depth of compression across the occupant body (seated or standing), and below 18 pounds-force (lbf) across skeletal locations of concern outlined in Figure 6.5-10—Anthropometric Locations for Blunt Trauma Limits & Skeletal Locations of Concern:
- Manubrium of the Sternum – Point 1
- Sternum (body) – Point 2
- Clavicle – Points 10 & 11
- Lateral aspect of the thorax – Points 22 & 23
- Vertebral Column – Points 40, 41, & 42
- Cervical Spine (C7) – Point 48
- Scapula – Point 31
- Spine – Point 39
- Acromion Process – Point 49
- Elbow (olecranon process) – Point 61
[Rationale: Meeting this requirement will enable performance, however the crewmember may experience minor discomfort that does not require first aid or medical attention. This requirement was initially developed for extraterrestrial surface transport vehicles but may apply to a wider range of systems (launch vehicles, elevators, landers etc.) if deemed applicable.
When addressing the blunt force limits, several considerations and general assumptions have been made. Further information can be found in NASA/TM-20220011974 Lunar Transport Vehicle Occupant Protection Requirements. These limits in Figure 6.5-12—Performance Blunt Force Maximum Allowable Depth of Compression Limits (Seated or Standing Vehicle Occupants), assume no intra-suit restraints other than a standard harness as seen in previous iterations of the EMU-style suit configurations. Additional occupant restraints in the form of inserts and suit modifications were not considered.
It is assumed that the vehicle shall provide proper foot restraints and back support in the proposed design. The vehicle occupant is also assumed to always remain in contact with supporting surfaces.
These limits cover all blunt forces imposed on the crewmember both directly by the system as well as indirectly by the system through a suit.
Design solutions are expected to contain energy attenuation to mitigate the blunt force the crewmember is subjected to.]
Figure 6.5-9—Performance Blunt Force Maximum Allowable Depth of Compression Limits (Seated or Standing Vehicle Occupants)
Figure 6.5-10—Anthropometric Locations for Blunt Trauma Limits & Skeletal Locations of Concern
6.6 Acoustics
This section establishes requirements to ensure an acceptable acoustic environment to preclude noise-related hearing loss and interference with communications and to support human performance. For technical requirements using sound to relay information, see section 10.5.1 Audio Systems, in this NASA Technical Standard. Table 6.6-1—Acoustic Limits for Launch, Entry, and Abort Phases, and Table 6.6-2—Acoustic Limits for On-Orbit, Lunar, and Planetary Operations Phase are summaries of the acoustic requirements listed in the following sections, referenced by requirement number.
To ensure that an integrated vehicle (including suit systems) meets the acoustic limits, it is necessary to develop an Acoustic Noise Control Plan that establishes the overall noise control strategy, acoustic limit allocations, acoustic testing, analyses, remedial action steps, schedule, and follow-up activities. This plan needs to be initially released early in the design cycle and then updated as new data and design information become available. The included acoustic limit allocations would define a set of allocated and sub-allocated acoustic limits for systems, subsystems, and hardware components so that the total contributions of all hardware will result in compliance with this NASA Technical Standard. As part of the Acoustic Noise Control Plan, it is best practice to verify acoustic requirements with test of the actual flight hardware. Modeling the acoustic environment of the vehicle with measured noise sources and propagation paths is best practice. Small design changes or even different part numbers of the same design such as with fans can change the acoustic level. Previous spaceflight experience has shown that without such a plan, it is difficult to develop an integrated system that can meet acoustic limits.
In this section there are cases that allow for or restrict the use of hearing protection to meet requirements. In cases where hearing protection is allowed, the phrase “at crewmember’s ear” is used. For cases where hearing protection cannot be used to satisfy these requirements, the phrase “at crew member’s head” is used. The allowance or restriction of hearing protection in each case is called out in the requirement or in the rationale.
Table 6.6-1—Acoustic Limits for Launch, Entry, and Abort Phases
Table 6.6-2—Acoustic Limits for On-Orbit, Lunar, and Planetary Operations Phase*
6.6.1 Acoustic Limits
6.6.1.1 Launch, Entry, and Abort Noise Exposure Limits
[V2 6073] During launch, entry, and abort operations, the noise exposure level (not including impulse noise) at the crewmember’s ear, calculated over any 24-hour period, shall be limited such that the noise dose (D) is ≤100:
N = the number of noise exposure events during the 24-hour period
Cn = the actual duration of the exposure event in minutes
Tn = the maximum noise exposure duration allowed, based on the specific sound level (Ln) of an exposure event in dBA, calculated using the following equation:
[Rationale: A noise dose of D = 100 is equivalent to an 8-hour, 85 dBA time-weighted average (TWA) using a 3 dB exchange rate. Equivalent noise exposure levels above 85 dBA for more than 8 hours have been shown to increase the risk of noise-induced hearing loss. The above formulae can be used to calculate the 24-hour noise exposure levels based on the 8-hour, 85 dBA criteria recommended by National Institute for Occupational Safety and Health (NIOSH), using the 3 dB exchange rate. The noise attenuation effectiveness of hearing protection, suit and helmet or communications headsets may be used to satisfy this requirement. Any planned use of hearing protection to satisfy this requirement is to be well documented and approved. Requirements established to meet this requirement are to be included in the Acoustic Noise Control Plan. The Acoustic Noise Control Plan allocates noise levels to individual components and is maintained to ensure that the total system meets the levels defined in this NASA Technical Standard.]
6.6.1.2 Ceiling Limit for Launch and Entry
[V2 6074] During launch and entry operations, the system shall limit the combined A-weighted sound levels (not including impulse noise) at the crewmembers’ ears to a maximum of 105 dBA.
[Rationale: Noise levels above 115 dBA have been shown to produce noise-induced hearing loss. In cases where audio communications are required, e.g., launch, entry, a 105 dBA limit is recommended to allow 10 dB of headroom for critical alarms and voice communications without exceeding the ultimate 115 dBA ceiling limit. The noise attenuation effectiveness of hearing protection or communications headsets may be used to satisfy this requirement; however, any planned use of hearing protection is to be well documented and approved. Program requirements established to meet this technical requirement are to be included in the Acoustic Noise Control Plan. This technical requirement does not apply to impulse noise sources.]
6.6.1.3 Ceiling Limit for Launch Abort
[V2 6075] During launch abort operations, the system shall limit the combined A-weighted sound levels (not including impulse noise) at the crewmembers’ ears to a maximum of 115 dBA.
[Rationale: Noise levels above 115 dBA have been shown to produce noise-induced hearing loss. In cases where no audio communications are required, e.g., during abort operations, there is no need to allow 10 dB of headroom for alarms and voice communications. The noise attenuation effectiveness of hearing protection or communications headsets may be used to satisfy this requirement; however, any planned use of hearing protection is to be well documented and approved.]
6.6.1.4 Launch, Entry, and Abort Impulse Noise Limits
[V2 6076] During launch, entry, and abort operations, impulse noise measured at the crewmember’s ear location shall be limited to less than 140 dB peak SPL.
[Rationale: A limit of 140 dB peak SPL for impulse noise prevents trauma to the hearing organs caused by impulse noise (OSHA 29 CFR 1910.95, Para 1910.95(b)(2) and WHO Environmental Health Criteria 12 – NOISE, Para 3.1.3). The noise attenuation effectiveness of hearing protection or communications headsets may be used to satisfy this requirement, however any planned use of hearing protection to satisfy this requirement is to be well documented and approved. Program requirements established to meet this technical requirement are to be included in the Acoustic Noise Control Plan.]
6.6.1.5 Hazardous Noise Limits for All Phases Except Launch, Entry, and Abort
[V2 6077] For off-nominal operations, broadcast communications, pressurization valve noise, and maintenance activities, the A-weighted sound level (excluding impulse noise and alarm signals) shall be less than 85 dBA (using fast exponential-time-averaging), regardless of the measured time interval; except in the case of pressurization valve noise, the noise attenuation effectiveness of hearing protection or communications headsets may not be used to satisfy this requirement.
[Rationale: The 85 dBA overall SPL defines the hazardous noise limit during all phases except launch, entry, and abort, at which action to reduce the noise level is to be taken so that interference with voice communications and alarms, as well as increased risk for hearing loss, does not occur. This is to help ensure that the habitable volume is safe. This is not intended for nominal hardware emissions but to limit the sound level of sources such as communications systems, depressurization valves, and levels that occur during planned off-nominal operations and maintenance activities. Nominal on-orbit acoustic levels are limited by requirements [V2 6078] Continuous Noise Limits and [V2 6080] Intermittent Noise Limits of this NASA Technical Standard. This requirement does not apply to impulse noise sources.]
6.6.1.6 24-Hour Noise Exposure Limits
[V2 6115] The noise exposure level (not including impulse noise) at the crewmember’s head, calculated over any 24-hour period, except during launch, entry, and abort operations, shall be limited such that the noise dose (D) is ≤100:
where:
N = the number of noise exposure events during the 24-hour period
Cn = the actual duration of the exposure event in minutes
Tn = the maximum noise exposure duration allowed, based on the specific sound level
(Ln) of an exposure event in dBA, calculated using the following equation:
[Rationale: A noise dose of D = 100 is equivalent to an 8-hour, 75 dBA time-weighted average (TWA) using a 3 dB exchange rate. Equivalent noise exposure levels above 75 dBA for more than 8 hours have been shown to increase the risk of noise-induced hearing loss. The above formulae can be used to calculate the 24-hour noise exposure levels based on the 8-hour, 75 dBA criterion recommended by the World Health Organization (WHO), using the 3 dB exchange rate, which corresponds to a 24-hour noise exposure level of 70 dBA or a 16-hour noise exposure level of 72 dBA. The noise attenuation effectiveness of hearing protection or communications headsets cannot be used to satisfy this requirement. Program requirements established to meet this technical requirement are to be included in the Acoustic Noise Control Plan. The Acoustic Noise Control Plan allocates noise levels to individual components and is maintained to ensure that the total noise exposure system meets the levels defined in this NASA Technical Standard.]
6.6.1.7 Continuous Noise Limits
[V2 6078] In spacecraft work areas, where good voice communications and habitability are required, SPLs of continuous noise (not including impulse or intermittent noise sources) shall be limited to the values given by the Noise Criterion (NC)-50 curve in Figure 6.6-1—NC Curves, and Table 6.6-3—Octave Band SPL Limits for Continuous Noise, dB re 20 µPa; hearing protection cannot be used to satisfy this requirement.
[Rationale: NC-50 limits noise levels within the habitable volume to allow adequate voice communications and habitability during mission operations. The noise limit at 16 kHz does not appear in Figure 6.6-1 but is given in Table 6.6-3. SPLs for continuous noise do not apply to alarms, communications, or noise experienced during maintenance activities. The corresponding spacesuit requirement is defined in requirement [V2 11009] Continuous Noise in Spacesuits, in this NASA Technical Standard.]
6.6.1.8 Crew Sleep Continuous Noise Limits
[V2 6079] For missions greater than 30 days, SPLs of continuous noise shall be limited to the values given by the NC-40 curve (see Figure 6.6-1—NC Curves, and Table 6.6-3—Octave Band SPL Limits for Continuous Noise, dB re 20 µPa) in crew quarters and sleep areas. Hearing protection cannot be used to satisfy this requirement.
[Rationale: For a crewmember to relax the auditory system, a quiet environment is to be provided during crew sleep; the NC-40 limit provides adequate auditory rest. The noise limit at 16 kHz does not appear in Figure 6.6-1—NC Curves but is given in Table 6.6-3—Octave Band SPL Limits for Continuous Noise. For missions 30 days or less in duration (short-duration missions), acoustic levels during sleep are limited by the requirement [V2 6078] Continuous Noise Limits (NC-50). Intermittent and impulse noise during sleep are controlled by the requirement [V2 6082] Annoyance Noise Limits for Crew Sleep. Some crewmembers find that using hearing protection causes sleep disturbance.]
Figure 6.6-1—NC Curves
Note: Corresponding A-weighted SPLs and speech interference levels (SILs) are given for reference only (Beranek and Vér, 1992). SIL (4) is Speech Interference Level, 4-band method.
Table 6.6-3—Octave Band SPL Limits for Continuous Noise, dB re 20 μPa
6.6.1.9 Intermittent Noise Limits
[V2 6080] For hardware items that operate for eight hours or less (i.e., intermittent noise), the maximum noise emissions (not including impulse noise), measured 0.6 m from the loudest hardware surface, shall be determined according to Table 6.6-4—Intermittent Noise A-Weighted SPL and Corresponding Operational Duration Limits for Any 24-hour Period (measured at 0.6 m distance from the source or closest distance to crew head, whichever is less). Hearing protection cannot be used to satisfy this requirement.
[Rationale: Table 6.6-4—Intermittent Noise A-Weighted SPL and Corresponding Operational Duration Limits for Any 24-hour Period limits crew exposure to intermittent noise levels of hardware items that are inherently noisy but that operate for short time periods. Intermittent sources can result in unacceptable noise levels, add to the overall crew noise exposure, impede communications, and cause disruption in crew rest/sleep.]
Table 6.6-4—Intermittent Noise A-Weighted SPL and Corresponding Operational Duration Limits for Any 24-Hour Period (measured at 0.6 m distance from the source or closest distance to crew head, whichever is less)
6.6.1.10 Alarm Maximum Sound Level Limit
[V2 6081] The maximum alarm signal A-weighted sound level shall be less than 95 dBA at the operating position of the intended receiver.
[Rationale: This allows broadcast alarm sound levels to exceed the 85 dBA hazard limit because of the need for alarm audibility. Also, alarms can be silenced at the crew’s discretion. This requirement does not apply during launch, entry, and abort (see [V2 6074] Ceiling Limit for Launch and Entry and [V2 6075] Ceiling Limit for Launch Abort), as these alarms will be provided by personal audio devices (see [V2 6106] Noise Limit for Personal Audio Devices).]
6.6.1.11 Annoyance Noise Limits for Crew Sleep
[V2 6082] With the exception of communications and alarms, the system shall limit impulse and intermittent noise levels at the crewmember’s head location to 10 dB above background noise levels during crew sleep periods. Hearing protection cannot be used to satisfy this requirement.
[Rationale: Impulse and intermittent noise is to be limited to 10 dB or less above the background noise to avoid waking crewmembers who are sleeping. Communications and alarms are not subject to this requirement.]
6.6.1.12 Impulse Noise Limit
[V2 6083] The system shall limit impulse noise measured at the crewmember’s head location to less than 140 dB peak SPL during all mission phases except launch, entry, and abort. Hearing protection cannot be used to satisfy this requirement.
[Rationale: A limit of 140-dB peak SPL for impulse noise prevents acoustic trauma (OSHA 29 CFR 1910.95, Para 1910.95(b)(2) and WHO Environmental Health Criteria 12 – NOISE, Para 3.1.3). Launch, Entry and Abort phases are exempted here as they allow for the use of hearing protection to satisfy a similar impulse noise limit requirement (see [V2 6076] Launch, Entry, and Abort Impulse Noise Limits).]
6.6.1.13 Narrow-Band Noise Limits
[V2 6084] The maximum SPL of narrow-band noise components and tones shall be limited to at least 10 dB less than the broadband SPL of the octave band that contains the component or tone.
[Rationale: Narrow-band noise component and tone levels are limited to 10 dB below the broadband level to prevent irritating and distracting noise conditions which could affect crew performance.]
6.6.1.14 Infrasonic Sound Pressure Limits
[V2 6085] Infrasonic SPLs, including frequencies from 1 to 20 Hz but not including impulse noise, shall be limited to less than 150 dB at the crewmember’s head location. Hearing protection cannot be used to satisfy this requirement.
[Rationale: The 150 dB limit for infrasonic noise levels in the frequency range from 1 to 20 Hz provides for health and well-being effects. Refer to ACGIH, Threshold Limit Values (TLVs®), Infrasound and Low-Frequency Sound, 2001.]
6.6.1.15 Noise Limit for Personal Audio Devices
[V2 6106] The system shall limit the maximum A-weighted sound level at the crewmember’s ear created by a personal audio device to 115 dBA or less when converted to a diffuse field.
[Rationale: Noise levels above 115 dBA have been shown to produce noise-induced hearing loss. Sound levels produced by personal audio devices are allowed to be at high levels to overcome the noise generated during launch and descent. A personal audio device may be an integrated part of the EVA helmet or an independent communication or listening headset but does not include cabin or broadcast speakers. OSHA identifies 115 dBA as the allowable ceiling for noise exposure limits. This ceiling limit is allowed as long as it does not result in the overall daily TWA exposure exceeding the limit of 85 dBA per requirement [V2 6073] Launch, Entry, and Abort Noise Exposure Limits. Testing is to be conducted to evaluate the upper limits of personal audio devices across all operable 1/3 O.B. frequencies using a swept sine signal driving the transducer acoustic output at the maximum specified device audio input level and the maximum audio output volume setting. Personal audio devices are to be determined compliant if the overall A-weighted levels are no greater than 115 dBA when converted to a diffuse field. This requirement is intended to be measured using an IEC 60318-4 compliant acoustic test fixture or head and torso simulator and converted to a diffuse field per IEEE Std 1652-2016.]
6.6.2 Acoustic and Noise Monitoring
6.6.2.1 Acoustic Monitoring
[V2 6087] Broadband and frequency-dependent SPLs shall be monitored and quantified as needed for crew health and safety.
[Rationale: Acoustic monitoring is needed to ensure that sound levels during the mission are below established limits for crew health and performance. As an example, periodically on ISS, the crew uses acoustic monitors to monitor their environment.]
6.6.2.2 Individual Noise Exposure Monitoring
[V2 6088] Noise exposure levels shall be monitored and quantified for each crewmember as needed for crew health and safety.
[Rationale: To protect the crew from excessive noise exposure, the noise exposure experienced by the crew is to be understood. This is critical to the protection of crew hearing and helps determine the degree of remedial actions needed, if any, including moving to a different environment, hardware shutdown, or proper implementation of countermeasures. As an example, periodically on ISS, the crew uses acoustic monitors to monitor their environment.]
6.7 Vibration
Limiting crew exposure to vibration is important for mission success. Excessive whole-body vibration can cause injury, fatigue, discomfort, and vision degradation, whereas the primary risk resulting from hand vibration is reduced fine motor control.
6.7.1 Whole Body Vibration
6.7.1.1 Vibration during Preflight
[V2 6089] The system shall limit vibration to the crew such that the frequency-weighted acceleration between 0.1 to 0.5 Hz in each of the X, Y, and Z axes is less than 0.05 g (0.5 m/s2) root mean square (RMS) for each 10-minute interval during prelaunch (when calculated in accordance with ISO 2631-1:1997(E), Mechanical Vibration and Shock – Evaluation of Human Exposure to Whole-Body Vibration – Part 1: General Requirements, Annex D, Equation D-1).
[Rationale: Low-frequency vibration, especially in the range between 0.1 and 0.5 Hz, has the potential to cause motion sickness over relatively short exposure periods. This may be encountered while the crew is in the vehicle during the prelaunch period, given that a tall vehicle stack may be susceptible to back-and-forth sway. Reducing the amount of sway will prevent the onset of motion sickness during the prelaunch phase. According to ISO 2631-1: 1997(E), Annex D, the percentage of unadapted adults who may vomit is equal to 1/3 motion sickness dose value. The value 0.05 g weighted RMS acceleration indicates that approximately 14% or one out of seven crewmembers may vomit. Although ISO 2631-1:1997(E) limits the acceleration measurement for assessing motion sickness to the vertical direction, this is based on the assumption that the human is in the seated upright posture. Since occupants of a vehicle are likely to be in the semi-supine posture, the requirement is applied to all three orthogonal axes (X, Y, and Z). The purpose of the 10-minute integration time is to constrain the deviations around the permitted average sway during a 2-hour prelaunch period.]
6.7.1.2 Vibration Exposures during Dynamic Phases of Flight
[V2 6090] The system shall limit vibration during dynamic phases of flight at interfaces that transmit vibration to the crew such that the vectorial sum of the X, Y, and Z accelerations between 0.5 and 80 Hz, calculated in 1-s intervals and weighted in accordance with ISO 26311:1997(E), is less than or equal to the levels for the accumulated durations in Table 6.7-1— Frequency-Weighted Vibration Limits by Exposure Time during Dynamic Phases of Flight, and Figure 6.7-1—Frequency-Weighted Vibration Limits by Exposure Time during Dynamic Phases of Flight.
[Rationale: Although there are limited data on the effects of high levels of vibration on health, especially during concurrent hypergravity acceleration, i.e., >1-g bias, internal organs and tissue structures may be damaged if the vibration amplitude goes over these time durations. This duration (under 10 minutes) is expected to bracket the vibration period during ascent and return. If the dynamic event exceeds this 10-minute duration, requirement [V2 6091] Long-Duration Vibration Exposure Limits for Health during Non-Sleep Phases of Mission must be used from the 10-minute point onward.
In accordance with ISO 2631-1, Section 6.3.1, vibration calculations are based on a running 1second time window. The limits depicted in Table 6.7-1—Frequency-Weighted Vibration Limits by Exposure Time during Dynamic Phases of Flight, and Figure 6.7-1—Frequency-Weighted Vibration Limits by Exposure Time during Dynamic Phases of Flight are to be applied as follows:
- The calculated frequency-weighted acceleration is to never exceed 6 m/s2 RMS (0.6 g RMS) for any 1-second time window.
- The calculated frequency-weighted acceleration may exceed 4 m/s2 RMS (0.4 g RMS) for no more than 60 non-overlapping 1-second time windows. These 1-second windows may be contiguous or noncontiguous.
- The calculated frequency-weighted acceleration for all of the remaining 1-second time windows during the maximum 10-minute per day dynamic phases of flight period is not to exceed 4 m/s2 RMS (0.4 g RMS). These 1-second windows may be contiguous or noncontiguous.]
Figure 6.7-1—Frequency-Weighted Vibration Limits by Exposure Time during Dynamic Phases of Flight
Table 6.7-1—Frequency-Weighted Vibration Limits by Exposure Time during Dynamic Phases of Flight
6.7.1.3 Long-Duration Vibration Dosage Limits for Health during Non-Sleep Phases of Mission
[V2 6091] The system shall limit vibration at interfaces that transmit vibration to the crew such that the accumulated dosage of the vectorial sum of the X, Y, and Z frequency-weighted accelerations, as computed according to ISO 2631-1:1997(E), does not exceed the minimum health guidance caution zone level defined in Figure 6.7-2—Long-Duration Vibration Dosage Limits for Health during Non-Sleep Phases of Mission.
[Rationale: Biodynamic and epidemiological research provides evidence of elevated health risk related to long-term exposure to high-intensity whole-body vibration. According to ISO 26311:1997(E) Annex B.3.1, “[f]or exposures below the [health guidance caution] zone, health effects have not been clearly documented and/or objectively observed.”
In accordance with ISO 2631-1:1997(E), Section 6.3.1, vibration calculations shall be based on a running 1-second time window. For this standard, the equivalent vibration dosage, aw,e, is computed per ISO 2631-1:1997(E), Eq. B.4 as:
for accumulated exposures up to or equal to 6 hours. For exposures exceeding 6 hours up to 24 hours, aw,e, is computed according to ISO 2631-1:1997(E), Eq. B.3 as:
where awi is frequency-weighted acceleration in m/s2 and Ti is the duration in seconds for the i-th time increment of the overall measurement or analysis period.
The allowable vibration dosage is depicted as a function of accumulated daily exposure time in Figure 6.7-2—Long Duration Vibration Dosage Limits for Health during Non-Sleep Phases of Mission. A vibration dosage of 3 m/s2 is permissible for up to 17 seconds of exposure during any 24-hour interval. For exposure durations between 17 seconds and 6 hours, the allowable zone is bounded per ISO 2631-1:1997(E), Eq. B.5, by:
where aw is the bounding vibration dosage accumulated as a function of exposure time, T (in seconds). For exposure durations between 6 and 24 hours, the zone is bounded by ISO 26311:1997(E), Eq. B.1:
where the bounding vibration dosage, a_w24 = 0.25 m/s2 , occurs at T_24 = 795,600 seconds (i.e., 24 hours). Finally, the frequency-weighted acceleration during any single 1-second window is not to exceed 3 m/s2.]
Figure 6.7-2—Long-Duration Vibration Dosage Limits for Health during Non-Sleep Phases of Mission
6.7.1.4 Vibration Exposure Limits during Sleep
[V2 6092] The system shall limit vibration to the crew such that the acceleration between 1.0 and 80 Hz in each of the X, Y, and Z axes, weighted in accordance with ISO 20283-5,
Mechanical Vibration—Measurement of Vibration on Ships; Part 5 – Guidelines for the Measurement, Evaluation and Reporting of Vibration with Regard to Habitability on Passenger and Merchant Ships, Annex A, is less than 0.01 g (0.1 m/s2) RMS for each two-minute interval during the crew sleep period.
[Rationale: For long-duration (approximately eight hours), smaller vibration exposure can adversely affect crew sleep. When addressing the vibration limits during sleep, consideration should be given to crewmember contact points (if any) with the system and whether system vibration can be transmitted at these points to the crewmember’s body.]
6.7.1.5 Vibration Limits for Performance
[V2 6093] The system shall ensure the appropriate level of crew task performance (e.g., motor control accuracy and precision, vision/readability, speech clarity, attentional focus) during vibration by evaluating task performance under all expected (nominal and off-nominal) vibration levels.
[Rationale: It is critical to ensure that the crew can perform mission tasks in the environment to which they will be exposed. For example, ascent and landing are typically accompanied by periods of significant vibration that can affect the crewmembers’ motor control and visual performance. Off-nominal conditions such as an aborted launch will expose crewmembers to challenging vibration levels that will limit their ability to perform functions such as reading display panels, turning knobs, activating switches, using touch screens and/or utilizing joystick controllers. Furthermore, by limiting exposure during crew task performance such that the acceleration in each of the frequencies between 0.5 and 80 Hz, calculated in 1-s intervals and weighted in accordance with ISO 2631-1:1997(E), Section 8.2.2.1, below 0.5 m/s2 (MIL-STD1472H 2020), one is likely to preserve appropriate performance. At this vibration level, conditions become “fairly uncomfortable” which can be expected to adversely impact performance. Furthermore, this level is consistent with NASA test data and visual performancebased requirement developed for NASA’s Orion crew vehicle. Thus, HITL testing with vibration becomes especially imperative for those situations where the 0.5 m/s2 level is anticipated to be exceeded.]
6.7.2 Hand Vibration
[V2 6094] The system, including tools, equipment, and processes, shall limit vibration to the crewmembers’ hands such that the accelerations, as computed according to ANSI/ASA S2.702006, Guide for the Measurement and Evaluation of Human Exposure to Vibration Transmitted to the Hand, do not exceed the Daily Exposure Action Value defined by ANSI/ASA S2.70-2006, Annex A, Figure A.1.
[Rationale: In accordance with ANSI/ASA S2.70-2006, Annex A.1.1, the Daily Exposure Action Value delineates the health risk threshold defined as “the dose of hand-transmitted vibration exposure sufficient to produce abnormal signs, symptoms, and laboratory findings in the vascular, bone or joint, neurological, or muscular systems of the hands and arms in some exposed individuals”.]
6.7.3 Extraterrestrial Surface Transport Vehicle Vibration Limits for Health and Performance
[V2 6160] The extraterrestrial surface transport vehicle (ESTV) system shall limit crewmember exposure to vibration such that the vectorial sum of the X, Y, and Z accelerations between 0.5 and 80 Hz, calculated in 1-s intervals and weighted in accordance with ISO 2631-1:1997(E), is less than or equal to the levels given in Figure 6.7-3—Extraterrestrial Surface Transport Vehicle Vibration Limits (Seated or Standing Vehicle Occupants) and Table 6.7-2—Extraterrestrial Surface Transport Vehicle Vibration Limits (Seated or Standing Vehicle Occupants), which specify the allowable accumulated duration (per 24-hour day) of vibration for both standing or seated crewmembers in all restraint conditions.
[Rationale: When addressing the sustained vibration limits, several considerations and general assumptions have been made. Further information can be found in NASA/TM-20220011974 Lunar Transport Vehicle Occupant Protection Requirements. These limits in Table 6.7-2—Extraterrestrial Surface Transport Vehicle Vibration Limits (Seated or Standing Vehicle Occupants) assume no intra-suit restraints other than a standard harness as seen in previous iterations of the EMU-style suit configurations. Additional occupant restraints in the form of inserts and suit modifications were not considered.
To define zones A, B, and C in Table 6.7-2, guidance from ISO 2631-1, MIL-STD-1472H, and MIL-STD-1472G, are leveraged. These documents outline specific health, performance and comfort thresholds based on cumulative exposure duration over a 24hr window. Overlaying the guidance from ISO 2631-1 and MIL-STD-1472 yields a plot with associated health and performance risk likelihoods and comfort levels (Figure 6.7-3—Extraterrestrial Surface Transport Vehicle Vibration Limits (Seated or Standing Vehicle Occupants).
Guidance from ISO 2631-1 is used to identify the health and performance consideration zone for short duration exposures (1s ≤ t ≤ 17s). For longer exposures (t > 17s), comfort guidance from MIL-STD-1472G and H is used. The identified time durations are cumulative dosage per 24hr window.
The ISO 2631-1 weighting function, Wd, and multiplying factor, k = 1.4, shall be used for the body x and y axes. The ISO 2631-1 weighting function, Wk, and multiplying factor, k = 1, shall be used for the body z axis. Vibration in multiple axes at a given measurement (or analysis) location shall be combined by taking the root sum of squares (RSS) of the weighted vibration in each body axis. If vibration couples to the vehicle occupant’s body at multiple locations, the weighted vibration computed as described shall be calculated by taking the RSS across all these locations. Following ISO 2631-1, a 1-s computation window is recommended for processing when all vibration frequency content of interest is at or above 1 Hz; a 2-s window may be used if there is significant frequency content between 0.5 and 1 Hz.
The limits depicted in Table 6.7-2— Extraterrestrial Surface Transport Vehicle Vibration Limits (Seated or Standing Vehicle Occupants), and Figure 6.7-3— Extraterrestrial Surface Transport Vehicle Vibration Limits (Seated or Standing Vehicle Occupants) are to be applied as follows:
- The calculated frequency-weighted acceleration shall never exceed 3 m/s2 RMS for any 1-second time window.
- The calculated frequency-weighted acceleration may exceed 0.5 m/s2 RMS for no more than 17 non-overlapping 1-second time windows. These 1-second windows may be contiguous or noncontiguous.
- The calculated frequency-weighted acceleration may exceed 0.315 m/s2 RMS for up to 216,000 non-overlapping 1-second windows (i.e., 6 hours). These 1-second windows may be contiguous or noncontiguous.
- The calculated frequency-weighted acceleration is not to exceed 0.315 m/s2 RMS for all remaining 1-second time windows during the 24-hour period. These 1-second windows may be contiguous or noncontiguous.
It is assumed that the vehicle will provide proper foot restraints and back support in the proposed design. The vehicle occupant is also assumed to always remain in contact with supporting surfaces.
These limits cover all vibrations imposed on the crewmember both directly by the ESTV as well as indirectly by the ESTV through the suit.
Design solutions are expected to contain energy attenuation to mitigate the vibration the crewmember is subjected to.]
Figure 6.7-3—Extraterrestrial Surface Transport Vehicle Vibration Limits (Seated or Standing Vehicle Occupants)
Table 6.7-2—Extraterrestrial Surface Transport Vehicle Vibration Limits (Seated or Standing Vehicle Occupants)
6.8 Radiation
6.8.1 Ionizing Radiation
Exploration of space requires traversal of environments that risk crew exposure to ionizing radiation such as charged particles, neutrons, X-rays and γ-rays. The ionizing radiation present in the mission envelope must be specified to aid in the evaluation and management of the exposure risk. The hazards from occupational exposure to ionizing radiation are mitigated through the application of the As Low As Reasonably Achievable (ALARA) principle during mission planning and vehicle design while the overall risk is limited by adherence to the permissible exposure limits (PELs). Application of the ALARA principle and adherence to PELs specified in Section 4.8 of NASA-STD-3001, Volume 1 protect the crew from acute effects and career-related cancer induced by radiation exposure. No level of increased exposure to ionizing radiation is considered safe and therefore the PELs are exclusion limits rather than tolerance values.
6.8.1.1 Radiation Environments
[V2 6098] The program shall specify the radiation environments to be used for design requirements and verification.
[Rationale: Defining the ionizing radiation environments across mission phases provides a starting point for planning and design that adheres to the ionizing radiation requirements set in Section 4.8 of NASA-STD-3001, Volume 1. The specified ionizing radiation environments drive derivative program requirements such as systems design and mission architecture. Definitions of radiation environments include, but are not limited to, specification of solar activity, types of ionizing radiation, energy spectra, and models used to generate the definitions. Example definitions of radiation environments can be found in Section 4.8 of NASA-STD-3001, Volume 1 and throughout SLS-SPEC-159, Revision I.]
6.8.1.2 Ionizing Radiation Protection Limit
[V2 6095] The program shall set system design requirements and operational constraints to prevent crewmembers from exceeding career space permissible exposure limits (PELs) as set forth in Section 4.8 of NASA-STD-3001, Volume 1.
[Rationale: Protection limits ensure that missions do no subject crew to ionizing radiation exposure beyond the PELs set in Section 4.8 of NASA-STD-3001, Volume 1. This requirement is imposed to limit the risk of long-term health effects of cancer.]
6.8.1.3 Intravehicular Area Monitoring of Space Radiation Exposure
[V2 6161] The program shall monitor the radiation exposure produced by galactic cosmic rays, solar energetic particles, trapped radiation, and neutrons in habitable volumes as referenced in Table 6.8-1—Space Radiation Monitoring Requirements Mission Location vs. Required Monitoring.
[Rationale: To characterize and manage crew exposure to ionizing radiation while ensuring the PELs are not exceeded, the ionizing radiation in habitable environments must be monitored throughout the course of a mission. Appropriate dose monitoring provides data on the radiation type, linear energy transfer (LET), intensity, and angles of incidence. Timepix-based instruments have been used by past programs to characterize the ionizing radiation inside crewed vehicles and to measure the dose and dose rates.]
Table 6.8-1—Space Radiation Monitoring Requirements Mission Location vs. Required Monitoring
6.8.1.4 Personal Monitoring of Space Radiation Exposure
[V2 6162] The program shall monitor the radiation exposure produced by galactic cosmic rays, solar energetic particles, and trapped radiation received by individual crew members as referenced in Table 6.8-1—Space Radiation Monitoring Requirements Mission Location vs. Required Monitoring.
[Rationale: Past and future career exposure to ionizing radiation of individual crew is considered during crew selection and mission planning. To ensure crew do not exceed PELs, the space radiation exposure of each crew member must be monitored on an individual basis as crew activity leads to varying absorbed dose. Individual dose monitoring must occur inside vehicles, habitats, and during extravehicular activity. These devices need to be compact and require minimal crew intervention for dose measurement to ensure crew compliance. The dose measurements from instruments fulfilling this requirement are entered into the crew medical records which are considered during crew selection.]
6.8.1.5 Area Monitoring of Radiation Exposure from Nuclear Technologies
[V2 6163] The program shall monitor the crew ionizing radiation exposure from nuclear technologies.
[Rationale: Nuclear technology integration into crewed vehicles and habitats poses an additional radiation exposure hazard beyond that of the space radiation environments. Ionizing radiation produced by nuclear technologies such as fission reactors and radioisotope thermoelectric generators must be monitored to ensure that the subcomponent of crew radiation exposure contributed by nuclear technologies does not exceed the limit set in [V1 4032] Crew Radiation Limits for Nuclear Technologies of NASA-STD-3001, Volume 1. This requirement may be fulfilled by dedicated monitors or instruments for intravehicular and extravehicular monitoring.]
6.8.1.6 Alerting of Elevated Exposure Rates
[V2 6164] The radiation monitoring system shall alert the crew and operations teams when radiation exposure rates exceed predefined thresholds.
[Rationale: The crew must be able to follow sheltering procedures planned in the event that dose rates exceed predefined thresholds. The crew must be alerted even if ground communication is disrupted or is unviable. Space weather events have the potential to expose crew to ionizing radiation that exceeds PELs during an event or through cumulative exposure over the course of a mission. Nominal communication mechanisms can be affected during space weather events requiring the monitoring systems to autonomously alert the crew or generate a signal to alarm through a connected system. The systems must alert operations teams if communication is available or upon being restored.]
6.8.1.7 External Space Weather Monitoring
[V2 6165] The program shall monitor the in-situ extravehicular space weather environment including the external exposure rates, electron flux spectra, and proton flux spectra.
[Rationale: Space weather events alter the typical mission radiation environments in ways that risk crew exposure to large doses of ionizing radiation before crew have adequate time to shelter. External radiation monitors can provide early warning to crew and ground personnel by detecting precursor particles that arrive ahead of the ionizing radiation that carries the bulk of exposure-related hazard. Space weather monitors that meet this requirement differ from crew dose monitors by their sensitivity to radiation typically absorbed by the shielding afforded by the vehicle or habitat. Space weather monitors must avoid saturation during significant storming such that exposure rates during such storms could be estimated. Historically significant space weather storming includes the August 1972 and October 1989 events.]
6.8.2 Non-Ionizing Radiation
Sources of non-ionizing radiation are present in spaceflight applications, and exposure is potentially hazardous. Astronaut occupational exposure to non-ionizing radiation is managed through mission architecture, system design, procedures and planning, and application of appropriate countermeasures. This NASA Technical Standard classifies non-ionizing radiation into four categories: radio frequency (RF) electromagnetic radiation, lasers, natural incoherent light, and artificial incoherent light.
6.8.2.1 RF Non-Ionizing Radiation Exposure Limits
[V2 6102] The system shall maintain the crew exposure to RF electromagnetic fields to or below the limits stated in Table 6.8-2—Maximum Permissible Exposure (MPE) to RF Electromagnetic Fields and shown graphically in Figure 6.8-1—RF Electromagnetic Field Exposure Limits.
[Rationale: Examples of devices that generate radio frequency radiation include, but are not limited to, antennas and wireless systems. These limits are modified from the C95.1-2005, Institute of Electrical and Electronic Engineers (IEEE). They are intended to establish exposure conditions for radio-frequency and microwave radiation to which it is believed that nearly all workers can be repeatedly exposed without injury. Modifications were made to the C95.1-2005 power density values to remove a safety margin that was added in the C95.1 standard to include the children population. This is not applicable to astronaut corp and resulted in the relaxation of the C95.1-2005 limits as per Figure 5.7.3.2.1-1, Occupational Exposure Limits for RadioFrequency Electromagnetic Fields.]
Table 6.8-2—Maximum Permissible Exposure (MPE) to RF Electromagnetic Fields (modified from IEEE C95.1-2005, lower tier)
(Illustrated to show whole-body resonance effects around 100 MHz) (modified from IEEE C95.1-2005, lower tier)
Figure 6.8-1—RF Electromagnetic Field Exposure Limits
6.8.2.2 Laser Exposure Limits
[V2 6103] The system shall maintain the crew ocular and dermal exposure to laser systems and the ocular exposure of the uncontrolled ground population to space lasers to or below the limits specified in ANSI Z136.1, 2014, American National Standard for Safe Use of Lasers, Table 5 (ocular) and Table 7 (dermal) without Personal Protective Equipment.
[Rationale: This requirement limits crew ocular and dermal exposure to both continuous and repetitively pulsed lasers to protect against eye and skin injury. The limits are adopted from the Laser Institute of America’s (LIA) publication ANSI Z136.1, 2014. The term laser system includes the laser, its housing, and controls. This requirement applies to laser systems utilized both internal and external to the vehicle. The safety analysis of all lasers will be carried out by ANSI Z136.1 methodology as specified in the verification requirement. In addition, this requirement limits uncontrolled ground population ocular exposure to space lasers. The limits are adopted from ANSI Z136.1, 2014. ANSI Z136.6, 2015, which may be used for guidance on laser hazard analysis methodology.]
6.8.2.3 Natural Sunlight Exposure Limits
[V2 6104] The system shall maintain the crew exposure to natural sunlight for spectral radiance or irradiance (as applicable) within wavelengths between 180 nm and 3000 nm, as noted in Table 6.8-3—Natural Sunlight Exposure Limits for Different Damage Mechanisms.
[Rationale: This requirement is intended to prevent ocular and dermal injury from sunlight exposure with wavelengths between 180 and 3000 nm. Any exposure should consider the entire window configuration of the incident radiation prior to its interaction with a crewmember’s body, including any concentration, diffusion, or filtering. The transmittance required for windows, visors, and other optical devices can be reconciled with protection from natural sunlight through the use of protective personal equipment, temporary filters, proper material selection, apertures, or other appropriate means. The sun subtends an angle of approximately 9 milliradians when observed from the Earth and is, therefore, considered a small source. The limits are based on the methodology given in the 2014 American Conference of Governmental Industrial Hygienists (ACGIH) standard, Threshold Limit Values® (TLVs) and Biological Exposure Indices® (BEIs), sections Light and Near-Infrared Radiation and Ultraviolet Radiation (2014 or newer). This requirement is applicable to both hatch and module windows.]
Table 6.8-3—Natural Sunlight Exposure Limits for Different Damage Mechanisms
6.8.2.4 Artificial Light Exposure Limits
[V2 6117] The system shall limit crew exposure from Visible, Infrared (IR), near-IR and Ultraviolet (UV) artificial light sources (180 nm to 3000 nm) at or below the threshold limit value (TLV) as calculated per ACGIH version 2022 or later.
[Rationale: This requirement is intended to prevent ocular injury and skin damage caused by exposure to ultraviolet radiation. Acceptable methods of containment include the use of lighttight structures and enclosures to fully contain the UV at the source and/or UV optical light obstruction by other means (e.g., screens, shields, filters) before reaching the crew to prevent exposure.
Grounded assessment of fault tolerance in avionics architecture is necessary to determine worstcase failure modes when verifying the requirement. For example, a credible failure mode of an electronic component could result in a crewmember being exposed to more than the nominal or safe amount of IR/UV radiation.]