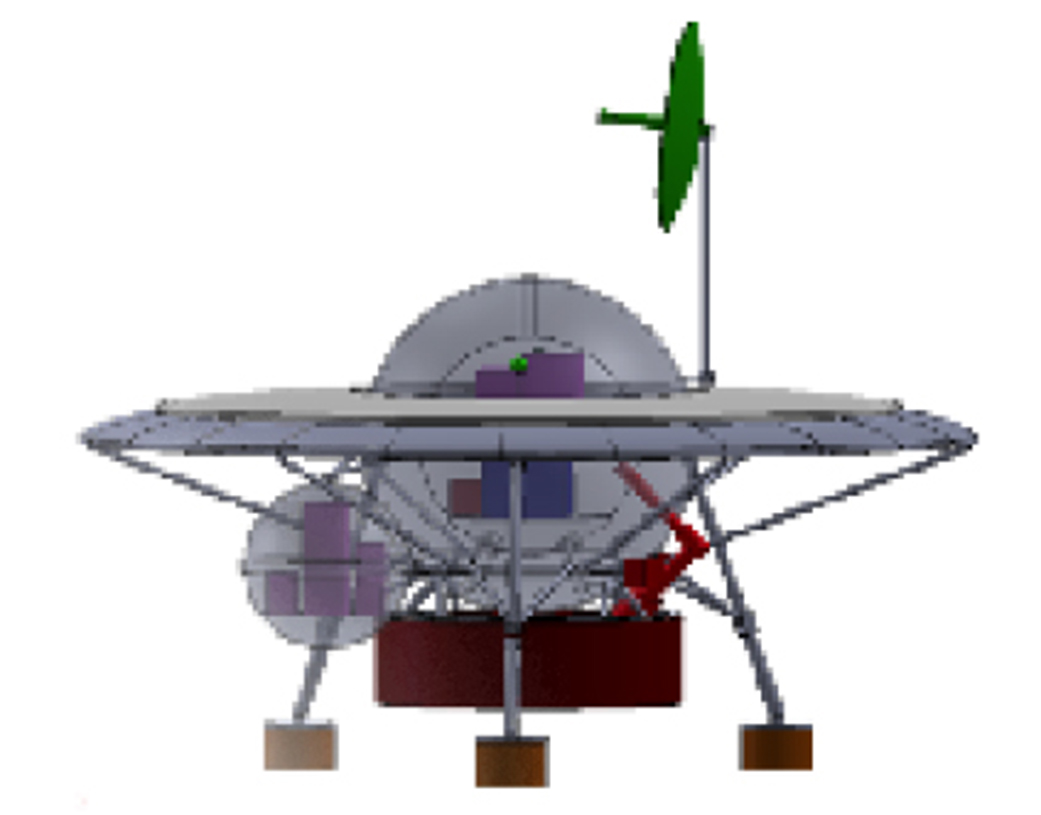
This ambitious photographic cartography of the Andromeda galaxy represents a new benchmark for precision studies of large spiral galaxies that dominate the universe’s population of over 100 billion galaxies. Never before have astronomers been able to see individual stars inside an external spiral galaxy over such a large contiguous area. Most of the stars in the universe live inside such majestic star cities, and this is the first data that reveal populations of stars in context to their home galaxy.
The Hubble Space Telescope is a project of international cooperation between NASA and the European Space Agency. NASA’s Goddard Space Flight Center in Greenbelt, Maryland, manages the telescope. The Space Telescope Science Institute (STScI) in Baltimore conducts Hubble science operations. STScI is operated for NASA by the Association of Universities for Research in Astronomy, Inc., in Washington.
Image Credit: NASA, ESA, J. Dalcanton, B.F. Williams, and L.C. Johnson (U. of Washington), the Panchromatic Hubble Andromeda Treasury (PHAT) team, and R. Gendler
Michael Paul
Pennsylvania State University
Description
Stored Chemical Energy Power Systems (SCEPS) have been used in U.S. Navy torpedoes for decades. This high-energy-density, high-power technology can be reliably stored for years. In Phase I we analyzed the applicability of SCEPS to in situ solar system exploration, looking to see if it could be adapted to power a lander sent to a target with no usable sunlight as an energy source. We developed a candidate mission to the surface of Venus, showing that SCEPS could be used for powering spacecraft and landers. The team compared it to conventional battery and Plutonium powered systems, both of which have deficiencies that are overcome by SCEPS. Our concept holds the promise of a power solution that could far exceed the operational capacity of existing batteries, allowing exciting exploration to continue despite the lack of available Plutonium. We propose to continue the research into applying SCEPS to exploration missions that can’t be powered by sunlight. In this study we will mature the Venus mission studied in Phase I. We will also expand our understanding of the usefulness of SCEPS to exploration of moons, comets, asteroids and other targets where sunlight is not sufficient to power the mission. We will engage with the leaders in science planning for small bodies, outer planets, and robotic missions to our own Moon and make a determination of the first, most high-impact use of SCEPS in space. An experiment will be performed to determine SCEPS performance when using CO2 as an oxidizer, approximating the in situ resource utilization of the Venusian atmosphere. Venus science goals will be revisited to prepare the Venus concept for the next level of study. Two key risks stand out. The first is our ability to scale down the power from current SCEPS implementation to levels more in family with spacecraft. Landed systems on Mars, for example, have had power levels on the order of hundreds of watts, far less than the many thousands of kilowatts that SCEPS provides for a U.S. Navy torpedo. The work proposed here would lead to better understanding of SCEPS operations at power levels appropriate to space exploration. The second risk is combustion with in situ resources. In the case of the ALIVE mission, the atmospheric CO2 is proposed as the oxidizer. The analysis performed in Phase I indicates that the reaction would give of the necessary heat to power the lander. The use of in situ resources has its benefits: in the case of the ALIVE mission it reduces the mass of consumables that would otherwise have to be included on launch day by hundreds of kilograms. In Phase II we seek experimental confirmation that this reaction can be initiated and sustained at the power levels required for such a lander. We see an opportunity to expand our understanding of the impact that SCEPS could have on solar system exploration. The sunless environment of Venus may indeed be explored through the use of SCEPS, but many cold, sunless regions may also benefit. Sending a SCEPS system to power a lander on the surface of Europa or the lakes or dunes of Titan may return substantial science that would be otherwise left unknown, or at least greatly delayed as the community works to solve the Plutonium-availability problem. We will develop a multi-variable model for SCEPS function and performance using advanced trade space visualization and exploration tools and techniques. The trade space will include the information gleaned from the stakeholders. The trade space tools will allow us to see the intersection of SCEPS capability and mission utility. The collective results of the study will be used to create a roadmap for further maturation of SCEPS for use in space. In Phase II we seek to expand the understanding of how best to target this technology and plan a path for development by developing a roadmap for TRL advancement of SCEPS in space that mirrors NASA’s solar system science goals in this decade.