Zipping through the sky 250 miles up is a shoebox-sized bundle of detectors and electronics named Dellingr. The namesake of the mythological Norse god of the dawn, Dellingr is among a new breed of spacecraft known as a CubeSat. These small satellites, measured in standardized 10-by-10-by-10 cubic centimeter units, weigh no more than a few pounds — bearing little resemblance to the larger, van-sized spacecraft such as the Hubble Telescope for which NASA is known. But SmallSats — which encompass a wide range of sizes, including CubeSats — are an increasingly valuable tool in the space scientist’s arsenal.
But CubeSats are still in their infancy, with mission success rates hovering near 50 percent. So, a team of scientists and engineers at NASA’s Goddard Space Flight Center in Greenbelt, Maryland, set out on a quest. Their goal? To build a more resilient CubeSat — one that could handle the inevitable on-flight mishaps that bedevil any spacecraft, without going kaput. They wanted a little CubeSat that could.
It was uncharted territory for them — an engineering exercise par excellence. The team was used to building big spacecraft, with the layers of process, analysis and testing that makes them reliable. Switching to CubeSats would require adapting or in some cases creating new processes and approaches, modifying organizational structures, all while working fast and on a limited budget. But it was an experiment worth trying, as the lessons they were sure to learn would benefit the entire CubeSat community. They got to work in 2014 and, after three years of development, Dellingr was ready to take flight.
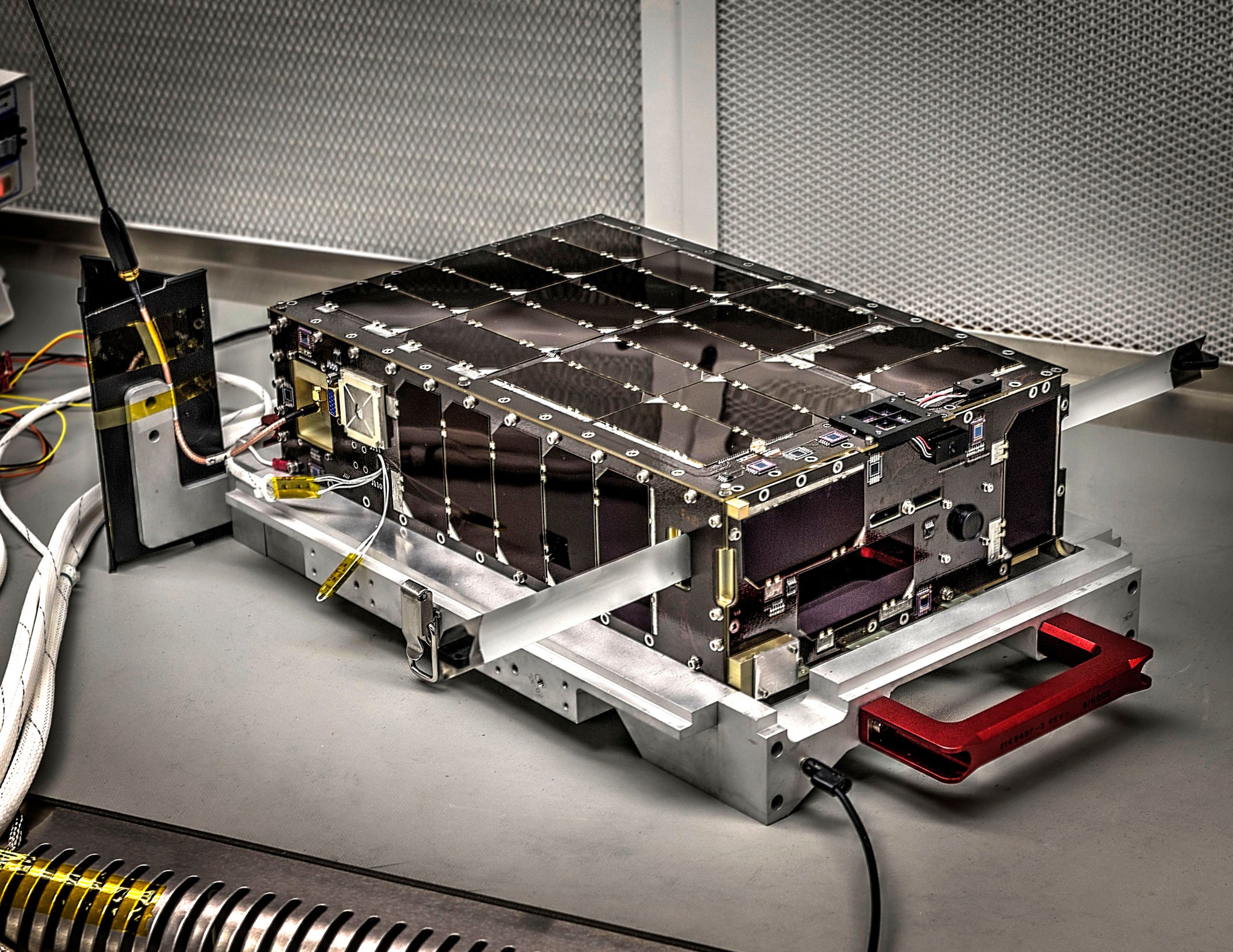
At the time of this writing Dellingr is flying overhead, transmitting valuable science and engineering data and working out its final kinks. But Dellingr’s journey has been far from smooth: The story of its launch, subsequent complications and successful fixes is a classic NASA tale of persistence and ingenuity.
Timeline
Aug. 14, 2017: Launch.
Dellingr launched aboard a Space-X Falcon 9 rocket on NASA’s CRS-12 mission to resupply the International Space Station. It sat as cargo for the next three months until deployment.
Nov. 20, 2017: Deployment from the ISS.
Just after noon EST, the Dellingr team watched a livestream from the International Space Station and cheered as Dellingr was released from the NanoRacks deployer.
Nov. 20, 2017: Seconds later.
As Dellingr escaped the ISS, the team’s excitement immediately turned to distress as they noticed small appendages sticking out from the spacecraft. A magnetometer, designed to measure Earth’s magnetic fields, and an antenna were already jutting out, despite having been programmed for a 30-minute delay period after deployment. Something was wrong.
Investigations revealed that the spacecraft had accidentally turned on during preparation for deployment, triggering the magnetometer and antenna while still inside the deployer — and running down the spacecraft’s power. Dellingr had been ejected into space with a dead battery.
Fortunately, like most CubeSats, Dellingr does not depend on propulsion to stay in orbit. Although “dead” in the air, the little satellite tumbled through space until its solar panels (which cover every surface of the spacecraft) sufficiently recharged the battery. Eight hours later, Dellingr made its first pass over its ground station at NASA’s Wallops Flight Facility in Wallops Island, Virginia. Data from the spacecraft indicated that it was fully functional, had automatically pointed at the Sun, and had a healthy battery charge. Despite the anomalous deployment, the spacecraft otherwise worked perfectly as designed.
Nov. 21 – 30, 2017: Outgassing.
In addition to two magnetometers designed to measure Earth’s magnetic fields, Dellingr carries an instrument called the Ion Neutral Mass Spectrometer, or INMS, which measures both ions and neutral particles in the atmosphere. The INMS instrument had never been fully validated in space. Showcasing what it could do was a major goal of the mission. However, before it could be turned on, INMS needed to complete the outgassing process — allowing harmful residue from Earth’s atmosphere to evaporate off of the spacecraft. Nothing to do but wait.
Nov. 30, 2017: Losing the Sun.
Dellingr determines its orientation in part by finding the Sun and tracking its position as it orbits Earth. By Nov. 30, the team had noticed that Dellingr was not holding lock on the Sun and appeared to be fidgeting around in space. The spacecraft’s orientation control system revved its reaction wheels — which spin in order to tilt the spacecraft one way or another — as it attempted to correct course.
But down on the ground, something didn’t look right. Dellingr has two Sun-pointers: a custom-built, high precision one, and a commercially purchased and flight-tested (albeit lower resolution) one. Only the custom Sun-pointer was returning wild-looking data. The spacecraft wasn’t wobbling — the custom Sun-pointer was malfunctioning.
Dellingr engineers uploaded quick-fix code to take it offline until they could figure out the root problem. But before they could do that, an even bigger issue arose.
Dec. 16, 2017: Loss of GPS.
Less than one month into orbit, Dellingr’s commercial GPS system abruptly reduced its power, dropped in temperature and stuttered to a stop. The GPS system was dead.
The loss of GPS meant that the team couldn’t precisely determine Dellingr’s position — and they also couldn’t determine its direction of movement, critical for proper orienting of the INMS instrument. INMS works like a snow plow, scooping up ions and neutral particles on the front end of the spacecraft as it flies through space.Without GPS, they couldn’t be sure the scoop was facing the right direction.
The team put Dellingr into minimal operational mode and began working on a plan for how to continue without GPS. By mid-January, they had formulated a plan and began making preparations to implement it. But, yet again, a new problem arose.
Jan. 27, 2018: The reset problem.
Spacecraft in orbit are always at risk of what are called single-event upsets that can scramble the spacecraft’s electrical signals — such as being struck by a high-speed cosmic ray or energetic particle from the Sun. To protect against single-event upsets, Dellingr was designed to complete a once-a-day, full-spacecraft reset to keep fresh; this reset had already protected the spacecraft on several occasions. In addition to the daily reset, Dellingr resets if it sees something wrong. While an occasional reset would be no cause for concern, in mid-January, Dellingr’s resets began triggering more often than they should. By Jan. 27 Dellingr was resetting every 63 seconds. Communications with the ground became impossible.
Jan. 28 – Feb. 5, 2018: Hatching a plan.
Dellingr was in a state of reset-induced paralysis. On the ground, the team had traced the reset problem to one line of code in a low-level device driver that involved the communication protocol used to control the reaction wheels, used for orienting the spacecraft. They needed to turn the reaction wheels off — but the constant resets prevented them from completing the commands to do so.
The team hatched a plan: On a pass over Dellingr’s ground station at Wallops Flight Facility, they would send a repeated series of commands to the spacecraft at a rapid pace, effectively jamming the computer so it never got far enough to reset. If they could jam it long enough, it would trigger a full power reset — the equivalent of unplugging the computer — buying them time to upload the solution and shut off the spacecraft’s reaction wheels. It was a long shot, but still their best bet.
Feb. 6, 2018: Back to business.
On a pass over Wallops on Feb. 6, the team tried the trick, and waited 90 minutes for the next pass when they could check the results. Shortly after, they received an email from the ground operator: “We confirm Dellingr back to business.” It worked.
Later that day the team turned on the INMS instrument, and the first real science measurements of the ions in the atmosphere with the novel INMS instrument were collected. The Dellingr team had validated the ion portion of the INMS instrument, achieving one of the major mission objectives.
Feb. 10 – March 5, 2018: Inching the wheels back on.
To solve the reset problem, Dellingr engineers had turned off the spacecraft’s reaction wheels — its primary tool for reorienting. As a result, it couldn’t remain steady and instead spun slowly throughout its orbit, collecting data only when the INMS instrument rotated through the front where it could scoop up particles. After some time, the team realized that the wheels could be used minimally — up to 24 hours at a time — without causing the resets. They developed a schedule to turn the wheels on at the beginning of each week, adjust orientation, and turn them off for the remainder. It worked — for a while.
March 6, 2018: The spin problem.
By March 6, it became clear that minimal use of the reaction wheels was not enough: Dellingr had entered an uncontrolled spin. Wobbling like a badly thrown football, Dellingr was spinning more than three times faster than its orientation control system could handle.
Over the next two months, the team worked on software solutions to control the rate of Dellingr’s spin without using the reaction wheels. The technique they settled on relied on the fact that magnets want to be aligned. The Earth is a giant magnet, and Dellingr contained three electromagnets that could be turned on and off by the spacecraft. By using Dellingr’s magnetometers as an orienting tool to sense Earth’s magnetic fields, and carefully timing when each onboard magnet was turned on, the spacecraft could take advantage of physics to slow down its spin, aligning the spacecraft with its direction of motion.
May 19 – 20, 2018: Dellingr is back on track.
After the third implementation of the de-spinning algorithm was uploaded and run, the spacecraft had stabilized. Dellingr had entered a very slow controlled spin, rolling like a wheel along its orbit. The INMS instrument now rotated to the front with a regular, predictable cadence.
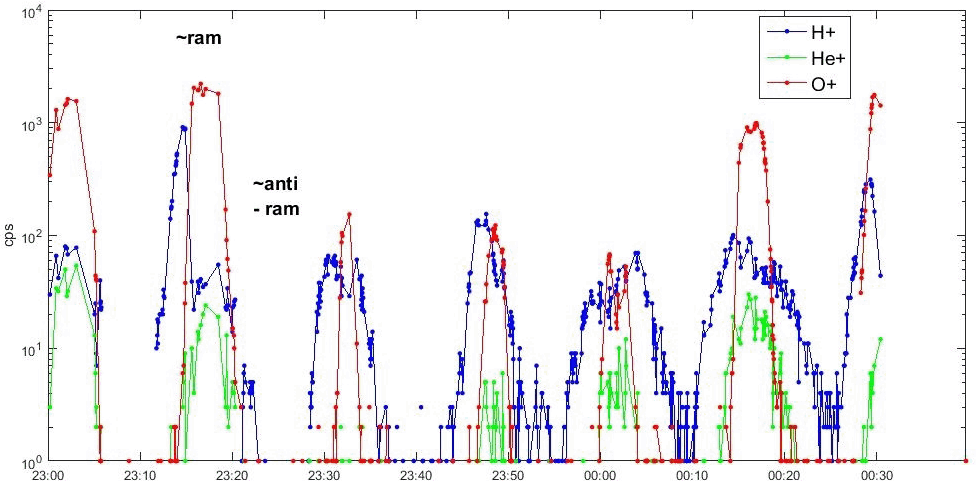
May 25, 2018: INMS is back.
With the spacecraft in a controlled spin, the INMS data went from a noisy mess to clear, periodic waves of data. After the spacecraft’s rotation was taken into account, the results were surprisingly clean, showing the detection of ionized hydrogen (H+), helium (He+) and oxygen (O+) in the atmosphere.
June 1, 2018: Aiming for neutrals.
Valid data from the INMS instrument — in ion mode — continues to stream in. Neutral mode, which is slightly more complicated, is still offline but is the focus of current efforts.
Oct. 5, 2018: Finding the Sun again.
With a large software upload, which took several weeks because of limitations with the CubeSat radio, the team restored full control of the reaction wheels, allowing Dellingr to maintain its orientation with respect to the Sun. The solar panels can now charge for maximum power generation as Dellingr slowly spins about that axis, collecting data. Almost a year after deployment, and after overcoming a rash of unexpected problems, the team had restored much of Dellingr’s functionality. Work on the neutral mode of the INMS instrument continues.
Dellingr already serves as a testament to the unique challenges associated with packing big science in a small box. Keeping it alive and running for this long was an important goal — a standard mission lifetime for CubeSats has not been validated, and Dellingr’s mission may help establish a benchmark. The team successfully achieved a resilient spacecraft mission, while still maintaining the low-cost aspect that is the hallmark of CubeSats. The value of the mission extends to others: papers are already being published outlining best practices learned from the mission, and these lessons learned have contributed to the successful proposals of three new Goddard CubeSat missions: petitSat, GTOSat and BurstCube.
The show’s not over — keep watching the skies to follow the story of this little CubeSat that could.
Background on CubeSats
CubeSats were pioneered by the California Polytechnic State University in 1999 and quickly became popular tools for students seeking to learn all aspects of spacecraft design and development. Today, they are a driving force that is opening up space research to public and private entities like never before. With off-the-shelf parts and a compact size that allows them to hitch a ride with other missions — they can, for example, be ejected from the International Space Station, up to six at a time — CubeSats have slashed the prohibitive price tag that satellite development once had, opening up doors to test new instruments as well as to create constellations of satellites working together. CubeSats can be flown in swarms, capturing simultaneous, multi-point measurements with identical instruments across a large area. Sampling entire physical systems in this way would drive forward our ability to understand the space environment around us, much the way multiple weather sensors help us understand weather systems.
Related:
- Press Release: “NASA Goddard Selects Michael Krainak as the IRAD Innovator of the Year; Team Award Bestowed on Dellingr Mission Team”
- Feature: “NASA’s Dellingr Team Awarded IRAD Team Award”
By Miles Hatfield
NASA’s Goddard Space Flight Center, Greenbelt, Md.